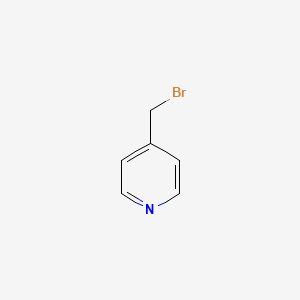
4-(Bromomethyl)pyridine
Overview
Description
4-(Bromomethyl)pyridine is an organic compound with the molecular formula C6H6BrN. It is a substituted pyridine where a bromomethyl group is attached to the fourth position of the pyridine ring. This compound is often used as an intermediate in organic synthesis due to its reactivity and versatility .
Mechanism of Action
Target of Action
4-(Bromomethyl)pyridine is a substituted pyridine . It is primarily used as a reagent in organic synthesis, and its specific targets can vary depending on the reaction it is involved in. For instance, it can react with 1,2-ethanediamine and 1,3-propanediamine to form the corresponding diamines .
Mode of Action
The mode of action of this compound is largely dependent on the specific reaction it is used in. In general, it acts as an electrophile, reacting with nucleophiles to form new bonds. For example, in the Suzuki–Miyaura cross-coupling reaction, it can participate in the transmetalation step, where it transfers its bromomethyl group to a palladium catalyst .
Biochemical Pathways
The specific biochemical pathways affected by this compound depend on the compounds it is reacted with. In the case of the Suzuki–Miyaura cross-coupling reaction, it contributes to the formation of new carbon-carbon bonds, which is a fundamental process in organic synthesis .
Result of Action
The result of this compound’s action is the formation of new organic compounds. For instance, it can be used in the preparation of various derivatives, such as 3-(4-pyridylmethyl)-2′,3′-di-O-oleyl-5′-O-(4,4′-dimethoxytriphenylmethyl)uridine and 1,4-bis(N-hexyl-4-pyridinium)butadiene diperchlorate .
Preparation Methods
Synthetic Routes and Reaction Conditions
4-(Bromomethyl)pyridine can be synthesized through several methods. One common method involves the bromination of 4-methylpyridine using N-bromosuccinimide (NBS) in the presence of a radical initiator such as benzoyl peroxide. The reaction typically occurs in a solvent like carbon tetrachloride at elevated temperatures .
Industrial Production Methods
In industrial settings, the production of this compound often involves continuous flow processes to ensure high yield and purity. The use of automated systems allows for precise control over reaction conditions, minimizing the formation of by-products and optimizing the efficiency of the process .
Chemical Reactions Analysis
Types of Reactions
4-(Bromomethyl)pyridine undergoes various types of chemical reactions, including:
Nucleophilic Substitution: The bromomethyl group can be substituted by nucleophiles such as amines, thiols, and alkoxides.
Oxidation: The compound can be oxidized to form pyridine carboxylic acids.
Reduction: Reduction reactions can convert the bromomethyl group to a methyl group.
Common Reagents and Conditions
Nucleophilic Substitution: Common reagents include sodium azide, potassium thiocyanate, and sodium methoxide. These reactions typically occur in polar aprotic solvents like dimethyl sulfoxide (DMSO) or acetonitrile.
Oxidation: Reagents such as potassium permanganate or chromium trioxide are used under acidic conditions.
Reduction: Catalytic hydrogenation using palladium on carbon (Pd/C) is a common method.
Major Products Formed
Nucleophilic Substitution: Products include azidomethylpyridine, thiocyanatomethylpyridine, and methoxymethylpyridine.
Oxidation: Products include pyridine-4-carboxylic acid.
Reduction: The major product is 4-methylpyridine.
Scientific Research Applications
4-(Bromomethyl)pyridine has a wide range of applications in scientific research:
Chemistry: It is used as a building block in the synthesis of more complex molecules, including pharmaceuticals and agrochemicals.
Biology: The compound is used in the modification of biomolecules for studying biological processes.
Medicine: It serves as an intermediate in the synthesis of drugs and diagnostic agents.
Industry: It is used in the production of polymers, dyes, and other industrial chemicals
Comparison with Similar Compounds
Similar Compounds
- 2-(Bromomethyl)pyridine
- 3-(Bromomethyl)pyridine
- 4-(Chloromethyl)pyridine
- 4-(Iodomethyl)pyridine
Uniqueness
4-(Bromomethyl)pyridine is unique due to its specific reactivity profile. The position of the bromomethyl group on the pyridine ring influences its reactivity and the types of reactions it can undergo. Compared to its analogs, this compound offers a balance of reactivity and stability, making it a valuable intermediate in organic synthesis .
Properties
IUPAC Name |
4-(bromomethyl)pyridine | |
---|---|---|
Source | PubChem | |
URL | https://pubchem.ncbi.nlm.nih.gov | |
Description | Data deposited in or computed by PubChem | |
InChI |
InChI=1S/C6H6BrN/c7-5-6-1-3-8-4-2-6/h1-4H,5H2 | |
Source | PubChem | |
URL | https://pubchem.ncbi.nlm.nih.gov | |
Description | Data deposited in or computed by PubChem | |
InChI Key |
KRLKXOLFFQWKPZ-UHFFFAOYSA-N | |
Source | PubChem | |
URL | https://pubchem.ncbi.nlm.nih.gov | |
Description | Data deposited in or computed by PubChem | |
Canonical SMILES |
C1=CN=CC=C1CBr | |
Source | PubChem | |
URL | https://pubchem.ncbi.nlm.nih.gov | |
Description | Data deposited in or computed by PubChem | |
Molecular Formula |
C6H6BrN | |
Source | PubChem | |
URL | https://pubchem.ncbi.nlm.nih.gov | |
Description | Data deposited in or computed by PubChem | |
DSSTOX Substance ID |
DTXSID40349061 | |
Record name | 4-(bromomethyl)pyridine | |
Source | EPA DSSTox | |
URL | https://comptox.epa.gov/dashboard/DTXSID40349061 | |
Description | DSSTox provides a high quality public chemistry resource for supporting improved predictive toxicology. | |
Molecular Weight |
172.02 g/mol | |
Source | PubChem | |
URL | https://pubchem.ncbi.nlm.nih.gov | |
Description | Data deposited in or computed by PubChem | |
CAS No. |
54751-01-8 | |
Record name | 4-(bromomethyl)pyridine | |
Source | EPA DSSTox | |
URL | https://comptox.epa.gov/dashboard/DTXSID40349061 | |
Description | DSSTox provides a high quality public chemistry resource for supporting improved predictive toxicology. | |
Retrosynthesis Analysis
AI-Powered Synthesis Planning: Our tool employs the Template_relevance Pistachio, Template_relevance Bkms_metabolic, Template_relevance Pistachio_ringbreaker, Template_relevance Reaxys, Template_relevance Reaxys_biocatalysis model, leveraging a vast database of chemical reactions to predict feasible synthetic routes.
One-Step Synthesis Focus: Specifically designed for one-step synthesis, it provides concise and direct routes for your target compounds, streamlining the synthesis process.
Accurate Predictions: Utilizing the extensive PISTACHIO, BKMS_METABOLIC, PISTACHIO_RINGBREAKER, REAXYS, REAXYS_BIOCATALYSIS database, our tool offers high-accuracy predictions, reflecting the latest in chemical research and data.
Strategy Settings
Precursor scoring | Relevance Heuristic |
---|---|
Min. plausibility | 0.01 |
Model | Template_relevance |
Template Set | Pistachio/Bkms_metabolic/Pistachio_ringbreaker/Reaxys/Reaxys_biocatalysis |
Top-N result to add to graph | 6 |
Feasible Synthetic Routes
Q1: Can 4-(bromomethyl)pyridine be utilized in the synthesis of novel catalytic materials?
A1: Yes, this compound can be used as a building block for synthesizing magnetic nanocatalysts. In a study by [], researchers successfully synthesized a novel magnetic nanocatalyst by reacting this compound hydrobromide with 3-(aminopropyl)triethoxysilane (APTES), followed by reaction with iron oxide nanoparticles and further modification with KBr and HIO4. This nanocatalyst, denoted as Fe3O4@PyHBr3, demonstrated catalytic activity in the selective oxidation of alcohols to aldehydes and ketones using H2O2 as an oxidant. Additionally, the Fe3O4@PyHBr3 nanocatalyst proved effective in catalyzing the trimethylsilylation and tetrahydropyranylation of alcohols [].
Q2: What is known about the stability of this compound in aqueous solutions?
A2: Research indicates that this compound undergoes hydrolysis in aqueous solutions, converting into the corresponding 4-(hydroxymethyl)pyridine []. This hydrolysis reaction follows first and second-order kinetics, with the rate being influenced by pH and temperature. At 60°C and an ionic strength (μ) of 0.15, the hydrolysis rates were meticulously studied across a pH range of 0.9 to 9.9 using buffer solutions. Through HPLC analysis and mathematical modeling, the researchers determined the rate constants for both the neutral this compound and its conjugated acid form []. The proposed mechanisms for this hydrolysis involve both unimolecular (SN1) and bimolecular (SN2) nucleophilic substitution pathways.
Q3: Has this compound been used in the development of metal complexes for material science applications?
A3: Yes, this compound, when reacted with divalent transition metal salts, can form mononuclear metal complexes. For instance, the reaction of this compound with iron(II) salts results in the creation of complexes with the general formula (2)center dot xMeNO(2), where L represents the 2,6-di(pyrazol-1-yl)-4-(bromomethyl) pyridine ligand []. These iron(II) complexes exhibit intriguing magnetic properties, namely thermal spin crossover behavior at elevated temperatures (around 320-340 K). It's noteworthy that the specific solvation state of these complexes significantly impacts their magnetic behavior, influencing the hysteresis loop's shape and width [].
Disclaimer and Information on In-Vitro Research Products
Please be aware that all articles and product information presented on BenchChem are intended solely for informational purposes. The products available for purchase on BenchChem are specifically designed for in-vitro studies, which are conducted outside of living organisms. In-vitro studies, derived from the Latin term "in glass," involve experiments performed in controlled laboratory settings using cells or tissues. It is important to note that these products are not categorized as medicines or drugs, and they have not received approval from the FDA for the prevention, treatment, or cure of any medical condition, ailment, or disease. We must emphasize that any form of bodily introduction of these products into humans or animals is strictly prohibited by law. It is essential to adhere to these guidelines to ensure compliance with legal and ethical standards in research and experimentation.