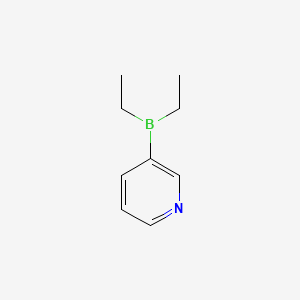
Diethyl(3-pyridyl)borane
Overview
Description
Diethyl(3-pyridyl)borane is an organoboron compound with the molecular formula C9H14BN. It is a white to almost white solid with a melting point of 172-175°C . This compound is slightly soluble in water and is primarily used as a reactant in various organic synthesis reactions .
Mechanism of Action
Target of Action
Diethyl(3-pyridyl)borane is primarily used as a reactant in the preparation of inhibitors of ataxia telangiectasia mutated and Rad3 related (ATR) protein kinase . The ATR protein kinase plays a crucial role in DNA damage response and is a potential target for anticancer agents .
Mode of Action
The compound interacts with its targets through a series of chemical reactions. It is used in N-Methylation reactions of the pyridyl group, Palladium catalyzed amination reactions, intramolecular Heck reactions, and coupling reactions . These reactions result in the formation of inhibitors that can potentially inhibit the ATR protein kinase .
Biochemical Pathways
This compound affects the biochemical pathway involved in the synthesis of trisubstituted pyrimidines from polyhalopyrimidines via Suzuki coupling . This pathway is significant in the development of anticancer agents targeting the ATR protein kinase .
Pharmacokinetics
It’s known that the compound is slightly soluble in water , which could impact its absorption and hence bioavailability
Result of Action
The primary result of the action of this compound is the formation of inhibitors of the ATR protein kinase . These inhibitors can potentially be used as anticancer agents, particularly for the treatment of prostate cancer .
Action Environment
The action of this compound is influenced by various environmental factors. For instance, the compound should be stored in an inert atmosphere at room temperature . Additionally, handling should be performed in a well-ventilated place, and dispersion of dust should be prevented . These conditions ensure the stability and efficacy of the compound.
Biochemical Analysis
Biochemical Properties
Diethyl(3-pyridyl)borane plays a significant role in biochemical reactions, particularly in the context of organic synthesis. It is involved in N-methylation reactions of the pyridyl group, palladium-catalyzed amination reactions, intramolecular Heck reactions, and coupling reactions . The compound interacts with enzymes such as ATR protein kinase, which is crucial for DNA damage response and repair mechanisms . These interactions are typically characterized by the formation of covalent bonds, which facilitate the inhibition of enzyme activity, thereby impacting cellular processes.
Cellular Effects
This compound has notable effects on various types of cells and cellular processes. It influences cell function by modulating cell signaling pathways, gene expression, and cellular metabolism. The compound’s interaction with ATR protein kinase, for instance, can lead to alterations in the DNA damage response, affecting cell cycle progression and apoptosis . Additionally, this compound may impact other cellular pathways, contributing to its potential as an anticancer agent.
Molecular Mechanism
The molecular mechanism of this compound involves its binding interactions with biomolecules, particularly enzymes like ATR protein kinase. By forming covalent bonds with these enzymes, the compound inhibits their activity, leading to downstream effects on cellular processes . This inhibition can result in changes in gene expression, as the DNA damage response pathways are altered. Additionally, this compound may interact with other proteins and biomolecules, further influencing cellular functions.
Temporal Effects in Laboratory Settings
In laboratory settings, the effects of this compound can change over time. The compound’s stability and degradation are critical factors that influence its long-term effects on cellular function. Studies have shown that this compound remains stable under specific conditions, but its activity may diminish over extended periods
Dosage Effects in Animal Models
The effects of this compound vary with different dosages in animal models. At lower doses, the compound may exhibit therapeutic effects, particularly in the context of cancer treatment . At higher doses, this compound can cause toxic or adverse effects, including skin irritation, eye irritation, and respiratory issues . These dosage-dependent effects highlight the importance of careful dosage optimization in therapeutic applications.
Metabolic Pathways
This compound is involved in various metabolic pathways, interacting with enzymes and cofactors that facilitate its biochemical activity. The compound’s role in N-methylation and palladium-catalyzed reactions underscores its involvement in complex metabolic processes . These interactions can influence metabolic flux and metabolite levels, contributing to the compound’s overall biochemical profile.
Transport and Distribution
Within cells and tissues, this compound is transported and distributed through interactions with transporters and binding proteins . These interactions determine the compound’s localization and accumulation in specific cellular compartments. The transport and distribution of this compound are critical for its biochemical activity and therapeutic potential.
Subcellular Localization
The subcellular localization of this compound is influenced by targeting signals and post-translational modifications that direct it to specific compartments or organelles . This localization is essential for the compound’s activity and function, as it determines the specific cellular processes that this compound can influence. Understanding the subcellular dynamics of this compound is crucial for optimizing its therapeutic applications.
Preparation Methods
Diethyl(3-pyridyl)borane can be synthesized through several methods. One common synthetic route involves the reaction of 3-bromopyridine with diethylmethoxyborane in the presence of n-butyllithium in dibutyl ether . The reaction is carried out at -78°C under a nitrogen atmosphere. After the reaction, the mixture is allowed to warm to room temperature, followed by the addition of water and brine. The organic layer is separated, dried over sodium sulfate, and concentrated. The resulting slurry is dissolved in isopropanol, cooled, and the product is isolated by filtration .
Chemical Reactions Analysis
Diethyl(3-pyridyl)borane undergoes various chemical reactions, including:
Oxidation: It can be oxidized to form boronic acids.
Reduction: It can be reduced to form boranes.
Substitution: It participates in substitution reactions, such as N-methylation of the pyridyl group.
Coupling Reactions: It is commonly used in Suzuki-Miyaura coupling reactions to form trisubstituted pyrimidines from polyhalopyrimidines.
Common reagents used in these reactions include palladium catalysts for coupling reactions and various oxidizing and reducing agents for other transformations. The major products formed from these reactions depend on the specific conditions and reagents used.
Scientific Research Applications
Diethyl(3-pyridyl)borane has several scientific research applications:
Comparison with Similar Compounds
Diethyl(3-pyridyl)borane can be compared with other similar organoboron compounds, such as:
Phenylboronic acid: Used in similar coupling reactions but has different reactivity due to the phenyl group.
Triethylborane: Another organoboron compound with different applications and reactivity.
Borane-pyridine complex: Used in reduction reactions but has different properties and uses compared to this compound .
This compound is unique due to its specific reactivity in coupling reactions and its role in the synthesis of biologically active compounds.
Properties
IUPAC Name |
diethyl(pyridin-3-yl)borane | |
---|---|---|
Source | PubChem | |
URL | https://pubchem.ncbi.nlm.nih.gov | |
Description | Data deposited in or computed by PubChem | |
InChI |
InChI=1S/C9H14BN/c1-3-10(4-2)9-6-5-7-11-8-9/h5-8H,3-4H2,1-2H3 | |
Source | PubChem | |
URL | https://pubchem.ncbi.nlm.nih.gov | |
Description | Data deposited in or computed by PubChem | |
InChI Key |
OJKBCQOJVMAHDX-UHFFFAOYSA-N | |
Source | PubChem | |
URL | https://pubchem.ncbi.nlm.nih.gov | |
Description | Data deposited in or computed by PubChem | |
Canonical SMILES |
B(CC)(CC)C1=CN=CC=C1 | |
Source | PubChem | |
URL | https://pubchem.ncbi.nlm.nih.gov | |
Description | Data deposited in or computed by PubChem | |
Molecular Formula |
C9H14BN | |
Source | PubChem | |
URL | https://pubchem.ncbi.nlm.nih.gov | |
Description | Data deposited in or computed by PubChem | |
DSSTOX Substance ID |
DTXSID00349087 | |
Record name | Diethyl(3-pyridyl)borane | |
Source | EPA DSSTox | |
URL | https://comptox.epa.gov/dashboard/DTXSID00349087 | |
Description | DSSTox provides a high quality public chemistry resource for supporting improved predictive toxicology. | |
Molecular Weight |
147.03 g/mol | |
Source | PubChem | |
URL | https://pubchem.ncbi.nlm.nih.gov | |
Description | Data deposited in or computed by PubChem | |
CAS No. |
89878-14-8 | |
Record name | 3-(Diethylboryl)pyridine | |
Source | CAS Common Chemistry | |
URL | https://commonchemistry.cas.org/detail?cas_rn=89878-14-8 | |
Description | CAS Common Chemistry is an open community resource for accessing chemical information. Nearly 500,000 chemical substances from CAS REGISTRY cover areas of community interest, including common and frequently regulated chemicals, and those relevant to high school and undergraduate chemistry classes. This chemical information, curated by our expert scientists, is provided in alignment with our mission as a division of the American Chemical Society. | |
Explanation | The data from CAS Common Chemistry is provided under a CC-BY-NC 4.0 license, unless otherwise stated. | |
Record name | 3-(Diethylboryl)pyridine | |
Source | ChemIDplus | |
URL | https://pubchem.ncbi.nlm.nih.gov/substance/?source=chemidplus&sourceid=0089878148 | |
Description | ChemIDplus is a free, web search system that provides access to the structure and nomenclature authority files used for the identification of chemical substances cited in National Library of Medicine (NLM) databases, including the TOXNET system. | |
Record name | Diethyl(3-pyridyl)borane | |
Source | EPA DSSTox | |
URL | https://comptox.epa.gov/dashboard/DTXSID00349087 | |
Description | DSSTox provides a high quality public chemistry resource for supporting improved predictive toxicology. | |
Record name | 3-(diethylboranyl)pyridine | |
Source | European Chemicals Agency (ECHA) | |
URL | https://echa.europa.eu/substance-information/-/substanceinfo/100.155.436 | |
Description | The European Chemicals Agency (ECHA) is an agency of the European Union which is the driving force among regulatory authorities in implementing the EU's groundbreaking chemicals legislation for the benefit of human health and the environment as well as for innovation and competitiveness. | |
Explanation | Use of the information, documents and data from the ECHA website is subject to the terms and conditions of this Legal Notice, and subject to other binding limitations provided for under applicable law, the information, documents and data made available on the ECHA website may be reproduced, distributed and/or used, totally or in part, for non-commercial purposes provided that ECHA is acknowledged as the source: "Source: European Chemicals Agency, http://echa.europa.eu/". Such acknowledgement must be included in each copy of the material. ECHA permits and encourages organisations and individuals to create links to the ECHA website under the following cumulative conditions: Links can only be made to webpages that provide a link to the Legal Notice page. | |
Record name | 3-(DIETHYLBORYL)PYRIDINE | |
Source | FDA Global Substance Registration System (GSRS) | |
URL | https://gsrs.ncats.nih.gov/ginas/app/beta/substances/3BC5KW7BC5 | |
Description | The FDA Global Substance Registration System (GSRS) enables the efficient and accurate exchange of information on what substances are in regulated products. Instead of relying on names, which vary across regulatory domains, countries, and regions, the GSRS knowledge base makes it possible for substances to be defined by standardized, scientific descriptions. | |
Explanation | Unless otherwise noted, the contents of the FDA website (www.fda.gov), both text and graphics, are not copyrighted. They are in the public domain and may be republished, reprinted and otherwise used freely by anyone without the need to obtain permission from FDA. Credit to the U.S. Food and Drug Administration as the source is appreciated but not required. | |
Synthesis routes and methods I
Procedure details
Synthesis routes and methods II
Procedure details
Retrosynthesis Analysis
AI-Powered Synthesis Planning: Our tool employs the Template_relevance Pistachio, Template_relevance Bkms_metabolic, Template_relevance Pistachio_ringbreaker, Template_relevance Reaxys, Template_relevance Reaxys_biocatalysis model, leveraging a vast database of chemical reactions to predict feasible synthetic routes.
One-Step Synthesis Focus: Specifically designed for one-step synthesis, it provides concise and direct routes for your target compounds, streamlining the synthesis process.
Accurate Predictions: Utilizing the extensive PISTACHIO, BKMS_METABOLIC, PISTACHIO_RINGBREAKER, REAXYS, REAXYS_BIOCATALYSIS database, our tool offers high-accuracy predictions, reflecting the latest in chemical research and data.
Strategy Settings
Precursor scoring | Relevance Heuristic |
---|---|
Min. plausibility | 0.01 |
Model | Template_relevance |
Template Set | Pistachio/Bkms_metabolic/Pistachio_ringbreaker/Reaxys/Reaxys_biocatalysis |
Top-N result to add to graph | 6 |
Feasible Synthetic Routes
Disclaimer and Information on In-Vitro Research Products
Please be aware that all articles and product information presented on BenchChem are intended solely for informational purposes. The products available for purchase on BenchChem are specifically designed for in-vitro studies, which are conducted outside of living organisms. In-vitro studies, derived from the Latin term "in glass," involve experiments performed in controlled laboratory settings using cells or tissues. It is important to note that these products are not categorized as medicines or drugs, and they have not received approval from the FDA for the prevention, treatment, or cure of any medical condition, ailment, or disease. We must emphasize that any form of bodily introduction of these products into humans or animals is strictly prohibited by law. It is essential to adhere to these guidelines to ensure compliance with legal and ethical standards in research and experimentation.