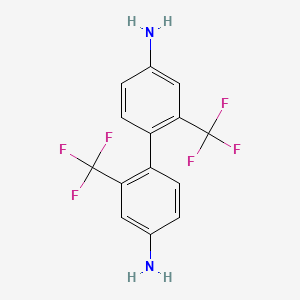
2,2'-Bis(trifluoromethyl)benzidine
Overview
Description
2,2’-Bis(trifluoromethyl)benzidine is a fluorinated benzidine derivative with the chemical formula C14H10F6N2. This compound is characterized by the presence of two trifluoromethyl groups at the 2 and 2’ positions on the benzidine structure. It is known for its rigidity and aromatic nature, making it a valuable building block in the synthesis of high-performance materials such as polyimides and metal-organic frameworks (MOFs) .
Mechanism of Action
Target of Action
The primary target of 2,2’-Bis(trifluoromethyl)benzidine is the π orbital on the fully conjugated polymer backbone . The compound interacts with these targets, influencing the electron delocalization of the π orbital .
Mode of Action
2,2’-Bis(trifluoromethyl)benzidine contains two fluoromethyl groups that have a strong electron-withdrawing effect . This effect reduces the electron delocalization in the π-orbitals of the fully conjugated polymer backbone .
Biochemical Pathways
The electron-withdrawing effect of 2,2’-Bis(trifluoromethyl)benzidine influences the chemical behavior of the compound, affecting various biochemical pathways . It contributes to the unique reactivity of the compound, enabling it to undergo different types of chemical reactions, including oxidation, reduction, and substitution reactions .
Pharmacokinetics
It is known that the compound is sparingly soluble in water but readily dissolves in various organic solvents . These properties may influence the compound’s absorption, distribution, metabolism, and excretion (ADME) properties, and thus its bioavailability.
Result of Action
The action of 2,2’-Bis(trifluoromethyl)benzidine results in significantly more transparent polyimide films compared to those with methyl substituents . This is due to the high transparency of the polyimides, which are used for solar cell encapsulation with metal-organic frameworks (MOFs) cluster light diffusers .
Biochemical Analysis
Biochemical Properties
2,2’-Bis(trifluoromethyl)benzidine plays a significant role in biochemical reactions due to the presence of trifluoromethyl groups, which influence its reactivity. This compound interacts with various enzymes, proteins, and other biomolecules. The trifluoromethyl groups have a strong electron-withdrawing effect, reducing the electron delocalization of the π orbital on the fully conjugated polymer backbone . This interaction leads to the formation of more transparent polyimide films compared to those with methyl substituents . Additionally, 2,2’-Bis(trifluoromethyl)benzidine is involved in the synthesis of high-performance resins, where it contributes to improved mechanical and dielectric properties .
Cellular Effects
2,2’-Bis(trifluoromethyl)benzidine has notable effects on various types of cells and cellular processes. It influences cell function by affecting cell signaling pathways, gene expression, and cellular metabolism. The compound’s strong electron-withdrawing effect reduces electron delocalization, leading to changes in the transparency of polyimide films . This property is particularly useful in applications such as solar cell encapsulation, where the compound enhances power conversion efficiency .
Molecular Mechanism
The molecular mechanism of 2,2’-Bis(trifluoromethyl)benzidine involves various binding interactions with biomolecules. The compound’s trifluoromethyl groups have a strong electron-withdrawing effect, which reduces the electron delocalization of the π orbital on the fully conjugated polymer backbone . This effect leads to the formation of more transparent polyimide films compared to those with methyl substituents . Additionally, the compound’s unique reactivity allows it to undergo different types of chemical reactions, including oxidation, reduction, and substitution reactions .
Temporal Effects in Laboratory Settings
In laboratory settings, the effects of 2,2’-Bis(trifluoromethyl)benzidine change over time. The compound exhibits good stability under normal conditions, showing resistance to light, heat, and moisture . Its long-term effects on cellular function have been observed in both in vitro and in vivo studies. The compound’s stability and degradation over time can influence its impact on cellular processes and overall function .
Dosage Effects in Animal Models
The effects of 2,2’-Bis(trifluoromethyl)benzidine vary with different dosages in animal models. At lower doses, the compound may exhibit beneficial properties, such as improved mechanical and dielectric properties in high-performance resins . At higher doses, the compound’s toxic and potentially carcinogenic nature becomes more pronounced, leading to adverse effects on health . It is crucial to carefully monitor and control the dosage of 2,2’-Bis(trifluoromethyl)benzidine in experimental settings to avoid toxic effects.
Metabolic Pathways
2,2’-Bis(trifluoromethyl)benzidine is involved in various metabolic pathways, interacting with enzymes and cofactors. The compound’s unique reactivity allows it to undergo different types of chemical reactions, including oxidation, reduction, and substitution reactions . These interactions can influence metabolic flux and metabolite levels, affecting the overall metabolic processes within cells .
Transport and Distribution
Within cells and tissues, 2,2’-Bis(trifluoromethyl)benzidine is transported and distributed through interactions with transporters and binding proteins. The compound’s strong electron-withdrawing effect reduces electron delocalization, influencing its localization and accumulation within specific cellular compartments . This property is particularly useful in applications such as solar cell encapsulation, where the compound enhances power conversion efficiency .
Subcellular Localization
The subcellular localization of 2,2’-Bis(trifluoromethyl)benzidine is influenced by its targeting signals and post-translational modifications. The compound’s unique reactivity and strong electron-withdrawing effect direct it to specific compartments or organelles within cells . This localization can affect the compound’s activity and function, influencing its overall impact on cellular processes .
Preparation Methods
The synthesis of 2,2’-Bis(trifluoromethyl)benzidine typically involves a two-step process:
Coupling Reaction: The initial step involves the coupling of bromo-5-nitro-trifluoromethyl toluene in a polar non-solvent under the catalytic action of a major catalyst and co-catalyst, along with the activation of a ligand. This reaction produces 2,2’-bis(trifluoromethyl)-4,4’-dinitro biphenyl.
Hydrogenation Reaction: The dinitro biphenyl compound is then subjected to hydrogenation in the presence of a catalyst and solvent, resulting in the formation of 2,2’-Bis(trifluoromethyl)benzidine .
Industrial production methods often focus on optimizing these reactions to improve yield and purity while minimizing energy consumption and catalyst usage.
Chemical Reactions Analysis
2,2’-Bis(trifluoromethyl)benzidine undergoes various chemical reactions, including:
Oxidation: This compound can be oxidized to form corresponding quinones.
Reduction: Reduction reactions typically involve the conversion of nitro groups to amino groups.
Substitution: The trifluoromethyl groups can participate in substitution reactions, often leading to the formation of derivatives with different functional groups.
Common reagents used in these reactions include hydrogen gas for reduction and various oxidizing agents for oxidation. The major products formed depend on the specific reaction conditions and reagents used .
Scientific Research Applications
2,2’-Bis(trifluoromethyl)benzidine has a wide range of applications in scientific research:
Chemistry: It is used as a monomer in the synthesis of polyimides, which are known for their thermal stability, chemical inertness, and excellent mechanical properties.
Biology: The compound’s unique properties make it useful in the development of biosensors and other analytical tools.
Industry: It is used in the production of high-performance materials such as MOFs and covalent organic frameworks (COFs), which have applications in gas storage, catalysis, and separation processes
Comparison with Similar Compounds
2,2’-Bis(trifluoromethyl)benzidine is unique due to the presence of trifluoromethyl groups, which impart distinct properties such as high thermal stability and chemical inertness. Similar compounds include:
4,4’-Diamino-2,2’-bis(trifluoromethyl)biphenyl: Another fluorinated benzidine derivative with similar applications in high-performance materials.
2,2’-Bis(trifluoromethyl)-4,4’-dinitrobiphenyl: A precursor in the synthesis of 2,2’-Bis(trifluoromethyl)benzidine, used in various chemical reactions
These compounds share some properties with 2,2’-Bis(trifluoromethyl)benzidine but differ in their specific applications and reactivity.
Properties
IUPAC Name |
4-[4-amino-2-(trifluoromethyl)phenyl]-3-(trifluoromethyl)aniline | |
---|---|---|
Source | PubChem | |
URL | https://pubchem.ncbi.nlm.nih.gov | |
Description | Data deposited in or computed by PubChem | |
InChI |
InChI=1S/C14H10F6N2/c15-13(16,17)11-5-7(21)1-3-9(11)10-4-2-8(22)6-12(10)14(18,19)20/h1-6H,21-22H2 | |
Source | PubChem | |
URL | https://pubchem.ncbi.nlm.nih.gov | |
Description | Data deposited in or computed by PubChem | |
InChI Key |
NVKGJHAQGWCWDI-UHFFFAOYSA-N | |
Source | PubChem | |
URL | https://pubchem.ncbi.nlm.nih.gov | |
Description | Data deposited in or computed by PubChem | |
Canonical SMILES |
C1=CC(=C(C=C1N)C(F)(F)F)C2=C(C=C(C=C2)N)C(F)(F)F | |
Source | PubChem | |
URL | https://pubchem.ncbi.nlm.nih.gov | |
Description | Data deposited in or computed by PubChem | |
Molecular Formula |
C14H10F6N2 | |
Source | PubChem | |
URL | https://pubchem.ncbi.nlm.nih.gov | |
Description | Data deposited in or computed by PubChem | |
DSSTOX Substance ID |
DTXSID70348007 | |
Record name | 2,2'-Bis(trifluoromethyl)benzidine | |
Source | EPA DSSTox | |
URL | https://comptox.epa.gov/dashboard/DTXSID70348007 | |
Description | DSSTox provides a high quality public chemistry resource for supporting improved predictive toxicology. | |
Molecular Weight |
320.23 g/mol | |
Source | PubChem | |
URL | https://pubchem.ncbi.nlm.nih.gov | |
Description | Data deposited in or computed by PubChem | |
CAS No. |
341-58-2 | |
Record name | 2,2'-Bis(trifluoromethyl)benzidine | |
Source | EPA DSSTox | |
URL | https://comptox.epa.gov/dashboard/DTXSID70348007 | |
Description | DSSTox provides a high quality public chemistry resource for supporting improved predictive toxicology. | |
Record name | [1,1'-Biphenyl]-4,4'-diamine, 2,2'-bis(trifluoromethyl)- | |
Source | European Chemicals Agency (ECHA) | |
URL | https://echa.europa.eu/information-on-chemicals | |
Description | The European Chemicals Agency (ECHA) is an agency of the European Union which is the driving force among regulatory authorities in implementing the EU's groundbreaking chemicals legislation for the benefit of human health and the environment as well as for innovation and competitiveness. | |
Explanation | Use of the information, documents and data from the ECHA website is subject to the terms and conditions of this Legal Notice, and subject to other binding limitations provided for under applicable law, the information, documents and data made available on the ECHA website may be reproduced, distributed and/or used, totally or in part, for non-commercial purposes provided that ECHA is acknowledged as the source: "Source: European Chemicals Agency, http://echa.europa.eu/". Such acknowledgement must be included in each copy of the material. ECHA permits and encourages organisations and individuals to create links to the ECHA website under the following cumulative conditions: Links can only be made to webpages that provide a link to the Legal Notice page. | |
Synthesis routes and methods I
Procedure details
Synthesis routes and methods II
Procedure details
Retrosynthesis Analysis
AI-Powered Synthesis Planning: Our tool employs the Template_relevance Pistachio, Template_relevance Bkms_metabolic, Template_relevance Pistachio_ringbreaker, Template_relevance Reaxys, Template_relevance Reaxys_biocatalysis model, leveraging a vast database of chemical reactions to predict feasible synthetic routes.
One-Step Synthesis Focus: Specifically designed for one-step synthesis, it provides concise and direct routes for your target compounds, streamlining the synthesis process.
Accurate Predictions: Utilizing the extensive PISTACHIO, BKMS_METABOLIC, PISTACHIO_RINGBREAKER, REAXYS, REAXYS_BIOCATALYSIS database, our tool offers high-accuracy predictions, reflecting the latest in chemical research and data.
Strategy Settings
Precursor scoring | Relevance Heuristic |
---|---|
Min. plausibility | 0.01 |
Model | Template_relevance |
Template Set | Pistachio/Bkms_metabolic/Pistachio_ringbreaker/Reaxys/Reaxys_biocatalysis |
Top-N result to add to graph | 6 |
Feasible Synthetic Routes
Q1: Why is TFMB a popular choice for synthesizing polyimides?
A1: TFMB's structure imparts desirable characteristics to the resulting polyimides. The presence of trifluoromethyl (-CF3) groups enhances solubility in organic solvents, enabling easier processing and film formation [, , , , ]. Additionally, the rigid biphenyl structure contributes to high glass transition temperatures (Tg) and excellent thermal stability, making TFMB-based polyimides suitable for high-temperature applications [, , , , , ].
Q2: Can TFMB-based polyimides be used in optical applications?
A2: Yes, incorporating TFMB can lead to colorless and transparent polyimide films, making them suitable for optical applications like flexible displays and optical waveguides. Researchers have achieved high transparency in the visible light range and low yellow index values by copolymerizing TFMB with specific dianhydrides like 4,4'-(hexafluoroisopropylidene)diphthalic anhydride (6FDA) [, , , , ].
Q3: How does TFMB influence the dielectric properties of polyimides?
A3: TFMB contributes to lowering the dielectric constant (k) of polyimides. The -CF3 groups reduce the polarizability of the polymer chains, resulting in lower dielectric constants desirable for microelectronic applications, especially as insulating layers in high-frequency circuits [, , , ].
Q4: Can the coefficient of thermal expansion (CTE) of polyimides be controlled using TFMB?
A4: Yes, incorporating TFMB can significantly impact the CTE of polyimides. Research indicates that TFMB, in conjunction with specific dianhydrides and processing techniques, can lead to polyimides with extremely low CTE values, even reaching negative CTEs [, , , , ]. This control over CTE is crucial for applications demanding dimensional stability over a wide temperature range, such as in microelectronics and aerospace.
Q5: How does TFMB influence the mechanical properties of polyimides?
A5: Polyimides synthesized with TFMB generally exhibit good mechanical properties. They typically display high tensile strength and modulus, making them suitable for applications requiring robust and durable films or coatings [, , ].
Q6: Are there any challenges in using TFMB for polyimide synthesis?
A6: While TFMB offers numerous advantages, challenges exist. For instance, polymerization with certain dianhydrides, like 2,3,6,7-naphthalenetetracarboxylic dianhydride (NTDA), can lead to very high Tg values, exceeding 400 °C, which might pose processing difficulties [].
Q7: What is the molecular formula and weight of TFMB?
A7: The molecular formula of TFMB is C14H10F6N2, and its molecular weight is 320.23 g/mol.
Q8: What spectroscopic techniques are typically used to characterize TFMB and its derived polymers?
A8: Common techniques include Fourier transform infrared spectroscopy (FTIR), nuclear magnetic resonance spectroscopy (NMR), and X-ray diffraction (XRD). FTIR helps identify functional groups, NMR provides structural information, and XRD reveals the crystalline or amorphous nature of the polymer [, , , ].
Q9: Have computational chemistry methods been employed to study TFMB and its polymers?
A9: Yes, molecular dynamics (MD) simulations have been used to investigate the structural evolution of TFMB-based polyimides during the pre-imidization process, offering insights into property changes at the molecular level [].
Q10: What is the significance of understanding the torsional angle between the phenyl rings in TFMB?
A10: The torsional angle influences the rigidity and packing of polymer chains, ultimately affecting properties like solubility and glass transition temperature [].
Q11: Is TFMB being explored for applications beyond polyimides?
A11: Yes, researchers are investigating TFMB for other applications. For instance, a fluorine-functionalized triazine-based porous organic polymer (F-POP) synthesized using TFMB displayed a remarkable ability to adsorb aflatoxins, indicating its potential in environmental remediation and food safety [].
Disclaimer and Information on In-Vitro Research Products
Please be aware that all articles and product information presented on BenchChem are intended solely for informational purposes. The products available for purchase on BenchChem are specifically designed for in-vitro studies, which are conducted outside of living organisms. In-vitro studies, derived from the Latin term "in glass," involve experiments performed in controlled laboratory settings using cells or tissues. It is important to note that these products are not categorized as medicines or drugs, and they have not received approval from the FDA for the prevention, treatment, or cure of any medical condition, ailment, or disease. We must emphasize that any form of bodily introduction of these products into humans or animals is strictly prohibited by law. It is essential to adhere to these guidelines to ensure compliance with legal and ethical standards in research and experimentation.