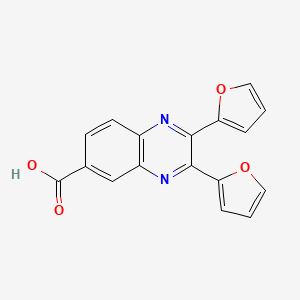
2,3-Di-furan-2-yl-quinoxaline-6-carboxylic acid
Overview
Description
2,3-Di-furan-2-yl-quinoxaline-6-carboxylic acid is an organic compound with the molecular formula C17H10N2O4. It is a derivative of quinoxaline, a bicyclic compound consisting of a benzene ring fused to a pyrazine ring. The presence of furan rings and a carboxylic acid group makes this compound particularly interesting for various chemical and biological applications .
Preparation Methods
Synthetic Routes and Reaction Conditions
The synthesis of 2,3-Di-furan-2-yl-quinoxaline-6-carboxylic acid typically involves the condensation of appropriate furan derivatives with quinoxaline precursors. One common method includes the reaction of 2-furylamine with 1,2-dicarbonyl compounds under acidic conditions to form the quinoxaline core, followed by carboxylation to introduce the carboxylic acid group .
Industrial Production Methods
The use of continuous flow reactors and automated synthesis platforms could enhance efficiency and scalability .
Chemical Reactions Analysis
Types of Reactions
2,3-Di-furan-2-yl-quinoxaline-6-carboxylic acid can undergo various chemical reactions, including:
Oxidation: The furan rings can be oxidized to form furanones.
Reduction: The quinoxaline core can be reduced to form dihydroquinoxaline derivatives.
Substitution: The carboxylic acid group can participate in esterification and amidation reactions.
Common Reagents and Conditions
Oxidation: Common oxidizing agents include potassium permanganate (KMnO4) and chromium trioxide (CrO3).
Reduction: Reducing agents such as sodium borohydride (NaBH4) and lithium aluminum hydride (LiAlH4) are typically used.
Major Products
Oxidation: Furanones
Reduction: Dihydroquinoxaline derivatives
Substitution: Esters and amides
Scientific Research Applications
2,3-Di-furan-2-yl-quinoxaline-6-carboxylic acid has a wide range of applications in scientific research:
Chemistry: Used as a building block for the synthesis of more complex organic molecules.
Biology: Investigated for its potential as a bioactive compound with antimicrobial and anticancer properties.
Medicine: Explored for its potential therapeutic effects, particularly in the development of new drugs.
Industry: Utilized in the production of specialty chemicals and materials.
Mechanism of Action
The mechanism of action of 2,3-Di-furan-2-yl-quinoxaline-6-carboxylic acid involves its interaction with various molecular targets. The furan rings and quinoxaline core can interact with enzymes and receptors, modulating their activity. The carboxylic acid group can form hydrogen bonds with biological molecules, enhancing binding affinity and specificity .
Comparison with Similar Compounds
Similar Compounds
2,3-Di-furan-2-yl-quinoxaline: Lacks the carboxylic acid group, affecting its reactivity and applications.
2,3-Di-thiophen-2-yl-quinoxaline-6-carboxylic acid: Contains thiophene rings instead of furan, altering its electronic properties and reactivity.
2,3-Di-furan-2-yl-pyridine-6-carboxylic acid: Has a pyridine ring instead of quinoxaline, impacting its biological activity.
Uniqueness
2,3-Di-furan-2-yl-quinoxaline-6-carboxylic acid is unique due to its combination of furan rings, quinoxaline core, and carboxylic acid group. This combination imparts distinct chemical and biological properties, making it a valuable compound for various applications .
Properties
IUPAC Name |
2,3-bis(furan-2-yl)quinoxaline-6-carboxylic acid | |
---|---|---|
Source | PubChem | |
URL | https://pubchem.ncbi.nlm.nih.gov | |
Description | Data deposited in or computed by PubChem | |
InChI |
InChI=1S/C17H10N2O4/c20-17(21)10-5-6-11-12(9-10)19-16(14-4-2-8-23-14)15(18-11)13-3-1-7-22-13/h1-9H,(H,20,21) | |
Source | PubChem | |
URL | https://pubchem.ncbi.nlm.nih.gov | |
Description | Data deposited in or computed by PubChem | |
InChI Key |
XDEQHYWMTHWMQF-UHFFFAOYSA-N | |
Source | PubChem | |
URL | https://pubchem.ncbi.nlm.nih.gov | |
Description | Data deposited in or computed by PubChem | |
Canonical SMILES |
C1=COC(=C1)C2=NC3=C(C=C(C=C3)C(=O)O)N=C2C4=CC=CO4 | |
Source | PubChem | |
URL | https://pubchem.ncbi.nlm.nih.gov | |
Description | Data deposited in or computed by PubChem | |
Molecular Formula |
C17H10N2O4 | |
Source | PubChem | |
URL | https://pubchem.ncbi.nlm.nih.gov | |
Description | Data deposited in or computed by PubChem | |
Molecular Weight |
306.27 g/mol | |
Source | PubChem | |
URL | https://pubchem.ncbi.nlm.nih.gov | |
Description | Data deposited in or computed by PubChem | |
Retrosynthesis Analysis
AI-Powered Synthesis Planning: Our tool employs the Template_relevance Pistachio, Template_relevance Bkms_metabolic, Template_relevance Pistachio_ringbreaker, Template_relevance Reaxys, Template_relevance Reaxys_biocatalysis model, leveraging a vast database of chemical reactions to predict feasible synthetic routes.
One-Step Synthesis Focus: Specifically designed for one-step synthesis, it provides concise and direct routes for your target compounds, streamlining the synthesis process.
Accurate Predictions: Utilizing the extensive PISTACHIO, BKMS_METABOLIC, PISTACHIO_RINGBREAKER, REAXYS, REAXYS_BIOCATALYSIS database, our tool offers high-accuracy predictions, reflecting the latest in chemical research and data.
Strategy Settings
Precursor scoring | Relevance Heuristic |
---|---|
Min. plausibility | 0.01 |
Model | Template_relevance |
Template Set | Pistachio/Bkms_metabolic/Pistachio_ringbreaker/Reaxys/Reaxys_biocatalysis |
Top-N result to add to graph | 6 |
Feasible Synthetic Routes
Disclaimer and Information on In-Vitro Research Products
Please be aware that all articles and product information presented on BenchChem are intended solely for informational purposes. The products available for purchase on BenchChem are specifically designed for in-vitro studies, which are conducted outside of living organisms. In-vitro studies, derived from the Latin term "in glass," involve experiments performed in controlled laboratory settings using cells or tissues. It is important to note that these products are not categorized as medicines or drugs, and they have not received approval from the FDA for the prevention, treatment, or cure of any medical condition, ailment, or disease. We must emphasize that any form of bodily introduction of these products into humans or animals is strictly prohibited by law. It is essential to adhere to these guidelines to ensure compliance with legal and ethical standards in research and experimentation.