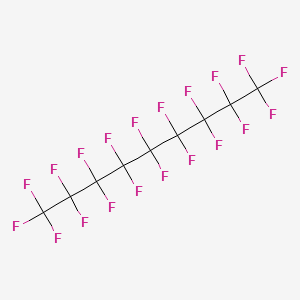
Perfluorononane
Overview
Description
Perfluorononane is a fully fluorinated organic compound with the molecular formula C₉F₂₀. It is a member of the perfluoroalkane family, where all hydrogen atoms in the nonane molecule are replaced by fluorine atoms. This compound is known for its high chemical stability, low surface tension, and unique physical properties, making it useful in various industrial and scientific applications .
Mechanism of Action
Target of Action
Perfluorononane is a common liquid perfluoro-n-alkane . It is primarily used as a heat transfer fluid in various industries . Its main purpose is to transfer heat efficiently in systems that require high thermal stability and low viscosity .
Mode of Action
The mechanism of action involves the chemical’s ability to absorb and release heat without undergoing any phase change . It is reported as an ideal oral contrast agent since it is biologically inert, immiscible with water, and also has a low viscosity and surface tension .
Biochemical Pathways
It is known that multiple pseudomonas species could degrade perfluorochemicals particularly perfluoroalkyl acids under aerobic condition . Acidimicrobium sp. degraded perfluoroalkyl acids anaerobically in the presence of electron donors .
Pharmacokinetics
It is known that this compound is a potent greenhouse gas and environment and health hazards posed by its toxic decomposition products have been evaluated .
Result of Action
This compound may be employed in the interface between active and buffer layer to improve the performance of polymer photovoltaic cells . It may also be employed as selective and specific markers for monitoring the gastric emptying and gastrointestinal (GI) transit times in mice and humans by using 27 Al and 19 F nuclear magnetic resonance (NMR) .
Action Environment
It is a potent greenhouse gas and environment and health hazards posed by its toxic decomposition products have been evaluated .
Biochemical Analysis
Biochemical Properties
Perfluorononane plays a role in biochemical reactions primarily due to its inert nature and unique electronic environment. It has been reported to interact with biomolecules such as proteins and enzymes through non-covalent interactions, including van der Waals forces and hydrophobic interactions. These interactions are typically weak and reversible, allowing this compound to act as a selective and specific marker for monitoring gastric emptying and gastrointestinal transit times in mice and humans . Additionally, this compound’s low surface tension and viscosity make it an ideal candidate for use in various biochemical assays and experiments.
Cellular Effects
The effects of this compound on various types of cells and cellular processes are primarily related to its inert nature. Due to its immiscibility with water, this compound does not readily interact with cellular membranes or intracellular components. It can influence cell function by acting as a contrast agent in imaging studies, allowing researchers to visualize and monitor cellular processes without interfering with normal cell function . This compound has also been shown to have minimal impact on cell signaling pathways, gene expression, and cellular metabolism, making it a valuable tool for studying cellular processes in a non-invasive manner.
Molecular Mechanism
At the molecular level, this compound exerts its effects through non-covalent interactions with biomolecules. These interactions include van der Waals forces and hydrophobic interactions, which allow this compound to bind to specific proteins and enzymes without altering their structure or function. This binding mechanism enables this compound to act as a selective marker in various biochemical assays and imaging studies . Additionally, this compound’s inert nature ensures that it does not interfere with enzyme activity or gene expression, making it a valuable tool for studying molecular mechanisms in a controlled environment.
Temporal Effects in Laboratory Settings
In laboratory settings, the effects of this compound have been observed to remain stable over time. The compound’s stability and resistance to degradation make it an ideal candidate for long-term studies and experiments. In both in vitro and in vivo studies, this compound has demonstrated consistent performance, with minimal changes in its effects on cellular function over extended periods . This stability ensures that this compound can be used reliably in various biochemical assays and imaging studies without the risk of degradation or loss of efficacy.
Dosage Effects in Animal Models
The effects of this compound in animal models have been studied at various dosages to determine its safety and efficacy. At low to moderate doses, this compound has been shown to have minimal toxic or adverse effects, making it a safe and effective tool for studying biochemical processes in vivo . At high doses, some threshold effects have been observed, including potential toxicity and adverse reactions. These findings highlight the importance of carefully controlling the dosage of this compound in animal studies to ensure accurate and reliable results.
Metabolic Pathways
This compound is involved in metabolic pathways primarily through its interactions with enzymes and cofactors. Due to its inert nature, this compound does not undergo significant metabolic transformations within the body. Instead, it acts as a stable marker for monitoring metabolic flux and metabolite levels in various biochemical assays . This stability ensures that this compound can be used reliably in metabolic studies without the risk of degradation or interference with normal metabolic processes.
Transport and Distribution
Within cells and tissues, this compound is transported and distributed primarily through passive diffusion. Its low viscosity and surface tension allow it to move freely within biological systems, interacting with transporters and binding proteins as needed . These interactions facilitate the localization and accumulation of this compound in specific tissues and compartments, making it an effective tool for studying transport and distribution processes in vivo.
Subcellular Localization
The subcellular localization of this compound is influenced by its inert nature and unique electronic environment. Due to its immiscibility with water, this compound does not readily interact with cellular membranes or intracellular components. It can be directed to specific compartments or organelles through targeting signals or post-translational modifications . This localization allows this compound to act as a selective marker for studying subcellular processes and interactions in a controlled environment.
Preparation Methods
Synthetic Routes and Reaction Conditions
Perfluorononane is typically synthesized through the fluorination of nonane. One common method involves the reaction of nonane with elemental fluorine in the presence of a catalyst such as cobalt trifluoride. This reaction is highly exothermic and requires careful control of temperature and pressure to ensure safety and yield optimization .
Industrial Production Methods
In industrial settings, this compound is produced using a continuous flow reactor where nonane is fluorinated under controlled conditions. The process involves multiple stages of fluorination and purification to achieve the desired product purity. The use of advanced fluorination techniques and equipment ensures high efficiency and scalability of production .
Chemical Reactions Analysis
Types of Reactions
Perfluorononane is highly inert due to the strong carbon-fluorine bonds, making it resistant to most chemical reactions. it can undergo certain types of reactions under specific conditions:
Oxidation: this compound can be oxidized using strong oxidizing agents, although this reaction is not commonly observed due to the compound’s stability.
Reduction: Reduction reactions are rare and typically require harsh conditions.
Substitution: Nucleophilic substitution reactions can occur, but they are generally slow and require strong nucleophiles
Common Reagents and Conditions
Oxidation: Strong oxidizing agents such as potassium permanganate or ozone.
Reduction: Lithium aluminum hydride or other strong reducing agents.
Substitution: Strong nucleophiles like sodium amide or potassium tert-butoxide
Major Products Formed
The major products formed from these reactions depend on the specific conditions and reagents used. For example, oxidation may yield perfluorinated carboxylic acids, while substitution reactions can introduce different functional groups into the this compound molecule .
Scientific Research Applications
Perfluorononane has a wide range of applications in scientific research due to its unique properties:
Chemistry: Used as a solvent for highly reactive species and in the synthesis of other fluorinated compounds.
Biology: Employed in biological studies as a contrast agent for imaging techniques such as magnetic resonance imaging (MRI) and ultrasonography.
Medicine: Investigated for use in artificial blood substitutes and oxygen carriers due to its high oxygen solubility.
Industry: Utilized in the electronics industry for cooling and as a dielectric fluid in high-voltage applications .
Comparison with Similar Compounds
Perfluorononane can be compared with other perfluorinated compounds such as perfluorooctane and perfluorodecalin. These compounds share similar properties, including high chemical stability and low surface tension. this compound is unique in its specific molecular structure, which provides distinct advantages in certain applications:
Perfluorooctane: Shorter carbon chain, used in similar applications but with different physical properties.
Perfluorodecalin: Cyclic structure, used in medical applications as an oxygen carrier .
Conclusion
This compound is a highly stable and inert compound with a wide range of applications in scientific research and industry Its unique properties make it valuable in various fields, from chemistry and biology to medicine and electronics
Biological Activity
Perfluorononane (PFNon) is a member of the perfluoroalkyl substances (PFAS) family, which are characterized by their carbon-fluorine bonds. This article explores the biological activity of PFNon, focusing on its effects on human health and the environment, as well as relevant case studies and research findings.
Overview of this compound
Chemical Structure and Properties:
- Chemical Formula: C9F18
- Molecular Weight: 414.06 g/mol
- Physical State: Colorless liquid
- Solubility: Highly hydrophobic and lipophobic, leading to bioaccumulation in living organisms.
PFNon is structurally similar to other PFAS, such as perfluorooctanoic acid (PFOA) and perfluorooctane sulfonate (PFOS), which have been extensively studied. However, research specifically targeting PFNon remains limited.
Biological Activity
1. Endocrine Disruption:
Research indicates that PFAS compounds can act as endocrine disruptors, affecting hormonal systems in both animals and humans. Although specific studies on PFNon are scarce, its structural similarities to PFOS suggest potential for similar biological activities.
- Case Study: A study evaluating PFOS indicated that it affects hormone receptor activity and steroidogenesis, leading to alterations in endocrine-related gene expression . Such findings raise concerns regarding PFNon's potential impact on endocrine functions.
2. Toxicological Effects:
PFAS compounds have been linked to various health issues, including liver toxicity, immune system dysfunction, and developmental abnormalities.
- Research Findings: A study involving primary hepatocytes showed that longer-chain perfluoroalkyl acids (including those similar to PFNon) could activate peroxisome proliferator-activated receptor alpha (PPARα), which plays a crucial role in lipid metabolism . This suggests that PFNon may also influence metabolic processes.
3. Neurotoxicity:
Evidence from studies on PFOS suggests that exposure can lead to neurodevelopmental issues. Given the structural similarities between PFOS and PFNon, it is plausible that PFNon could exhibit neurotoxic effects as well.
- Case Study: In a study with Xenopus laevis embryos exposed to PFOS, significant developmental disruptions were observed at various concentrations . While direct studies on PFNon are lacking, these findings highlight the need for further investigation into its neurotoxic potential.
Data Tables
Biological Activity | PFNon | PFOS | PFOA |
---|---|---|---|
Endocrine disruption | Unknown | Yes | Yes |
Liver toxicity | Unknown | Yes | Yes |
Neurodevelopmental effects | Unknown | Yes | Yes |
Immune system effects | Unknown | Yes | Yes |
Case Studies
-
Environmental Impact Assessment:
A site contaminated with various PFAS, including PFOA and PFOS, demonstrated significant remediation challenges due to their persistence in the environment. The application of innovative remediation techniques has shown promise in reducing contaminant levels over extended periods . -
Human Health Studies:
Epidemiological studies linking PFAS exposure to adverse health outcomes provide indirect evidence of potential risks associated with compounds like PFNon. The long-term health effects observed in populations exposed to high levels of PFOS and PFOA may also apply to similar compounds .
Properties
IUPAC Name |
1,1,1,2,2,3,3,4,4,5,5,6,6,7,7,8,8,9,9,9-icosafluorononane | |
---|---|---|
Source | PubChem | |
URL | https://pubchem.ncbi.nlm.nih.gov | |
Description | Data deposited in or computed by PubChem | |
InChI |
InChI=1S/C9F20/c10-1(11,2(12,13)4(16,17)6(20,21)8(24,25)26)3(14,15)5(18,19)7(22,23)9(27,28)29 | |
Source | PubChem | |
URL | https://pubchem.ncbi.nlm.nih.gov | |
Description | Data deposited in or computed by PubChem | |
InChI Key |
UVWPNDVAQBNQBG-UHFFFAOYSA-N | |
Source | PubChem | |
URL | https://pubchem.ncbi.nlm.nih.gov | |
Description | Data deposited in or computed by PubChem | |
Canonical SMILES |
C(C(C(C(C(F)(F)F)(F)F)(F)F)(F)F)(C(C(C(C(F)(F)F)(F)F)(F)F)(F)F)(F)F | |
Source | PubChem | |
URL | https://pubchem.ncbi.nlm.nih.gov | |
Description | Data deposited in or computed by PubChem | |
Molecular Formula |
C9F20 | |
Record name | Nonane, 1,1,1,2,2,3,3,4,4,5,5,6,6,7,7,8,8,9,9,9-eicosafluoro- | |
Source | NORMAN Suspect List Exchange | |
Description | The NORMAN network enhances the exchange of information on emerging environmental substances, and encourages the validation and harmonisation of common measurement methods and monitoring tools so that the requirements of risk assessors and risk managers can be better met. The NORMAN Suspect List Exchange (NORMAN-SLE) is a central access point to find suspect lists relevant for various environmental monitoring questions, described in DOI:10.1186/s12302-022-00680-6 | |
Explanation | Data: CC-BY 4.0; Code (hosted by ECI, LCSB): Artistic-2.0 | |
Source | PubChem | |
URL | https://pubchem.ncbi.nlm.nih.gov | |
Description | Data deposited in or computed by PubChem | |
DSSTOX Substance ID |
DTXSID60334479 | |
Record name | Perfluorononane | |
Source | EPA DSSTox | |
URL | https://comptox.epa.gov/dashboard/DTXSID60334479 | |
Description | DSSTox provides a high quality public chemistry resource for supporting improved predictive toxicology. | |
Molecular Weight |
488.06 g/mol | |
Source | PubChem | |
URL | https://pubchem.ncbi.nlm.nih.gov | |
Description | Data deposited in or computed by PubChem | |
CAS No. |
375-96-2 | |
Record name | Perfluorononane | |
Source | EPA DSSTox | |
URL | https://comptox.epa.gov/dashboard/DTXSID60334479 | |
Description | DSSTox provides a high quality public chemistry resource for supporting improved predictive toxicology. | |
Record name | Perfluorononane | |
Source | European Chemicals Agency (ECHA) | |
URL | https://echa.europa.eu/information-on-chemicals | |
Description | The European Chemicals Agency (ECHA) is an agency of the European Union which is the driving force among regulatory authorities in implementing the EU's groundbreaking chemicals legislation for the benefit of human health and the environment as well as for innovation and competitiveness. | |
Explanation | Use of the information, documents and data from the ECHA website is subject to the terms and conditions of this Legal Notice, and subject to other binding limitations provided for under applicable law, the information, documents and data made available on the ECHA website may be reproduced, distributed and/or used, totally or in part, for non-commercial purposes provided that ECHA is acknowledged as the source: "Source: European Chemicals Agency, http://echa.europa.eu/". Such acknowledgement must be included in each copy of the material. ECHA permits and encourages organisations and individuals to create links to the ECHA website under the following cumulative conditions: Links can only be made to webpages that provide a link to the Legal Notice page. | |
Retrosynthesis Analysis
AI-Powered Synthesis Planning: Our tool employs the Template_relevance Pistachio, Template_relevance Bkms_metabolic, Template_relevance Pistachio_ringbreaker, Template_relevance Reaxys, Template_relevance Reaxys_biocatalysis model, leveraging a vast database of chemical reactions to predict feasible synthetic routes.
One-Step Synthesis Focus: Specifically designed for one-step synthesis, it provides concise and direct routes for your target compounds, streamlining the synthesis process.
Accurate Predictions: Utilizing the extensive PISTACHIO, BKMS_METABOLIC, PISTACHIO_RINGBREAKER, REAXYS, REAXYS_BIOCATALYSIS database, our tool offers high-accuracy predictions, reflecting the latest in chemical research and data.
Strategy Settings
Precursor scoring | Relevance Heuristic |
---|---|
Min. plausibility | 0.01 |
Model | Template_relevance |
Template Set | Pistachio/Bkms_metabolic/Pistachio_ringbreaker/Reaxys/Reaxys_biocatalysis |
Top-N result to add to graph | 6 |
Feasible Synthetic Routes
Disclaimer and Information on In-Vitro Research Products
Please be aware that all articles and product information presented on BenchChem are intended solely for informational purposes. The products available for purchase on BenchChem are specifically designed for in-vitro studies, which are conducted outside of living organisms. In-vitro studies, derived from the Latin term "in glass," involve experiments performed in controlled laboratory settings using cells or tissues. It is important to note that these products are not categorized as medicines or drugs, and they have not received approval from the FDA for the prevention, treatment, or cure of any medical condition, ailment, or disease. We must emphasize that any form of bodily introduction of these products into humans or animals is strictly prohibited by law. It is essential to adhere to these guidelines to ensure compliance with legal and ethical standards in research and experimentation.