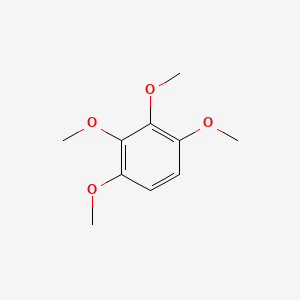
1,2,3,4-Tetramethoxybenzene
Overview
Description
1,2,3,4-Tetramethoxybenzene: is an organic compound with the molecular formula C10H14O4 . It is a derivative of benzene, where four methoxy groups are attached to the benzene ring at the 1, 2, 3, and 4 positions. This compound is known for its aromatic properties and is used in various chemical and industrial applications .
Scientific Research Applications
1,2,3,4-Tetramethoxybenzene has several applications in scientific research:
Chemistry: It is used as a building block for the synthesis of more complex organic molecules.
Industry: It is used in the production of dyes, fragrances, and other aromatic compounds.
Preparation Methods
Synthetic Routes and Reaction Conditions: 1,2,3,4-Tetramethoxybenzene can be synthesized through the methylation of 1,2,3,4-tetrahydroxybenzene. The methylation process typically involves the use of methyl iodide (CH3I) and a base such as potassium carbonate (K2CO3) in a suitable solvent like acetone. The reaction is carried out under reflux conditions to ensure complete methylation of the hydroxyl groups .
Industrial Production Methods: In an industrial setting, the production of this compound may involve the use of more efficient and scalable methods. One such method includes the use of dimethyl sulfate (DMS) as the methylating agent in the presence of a base like sodium hydroxide (NaOH). The reaction is conducted in a solvent such as dimethylformamide (DMF) at elevated temperatures to achieve high yields .
Chemical Reactions Analysis
Types of Reactions: 1,2,3,4-Tetramethoxybenzene undergoes various chemical reactions, including:
Oxidation: The compound can be oxidized to form quinones using oxidizing agents like potassium permanganate (KMnO4) or chromium trioxide (CrO3).
Reduction: Reduction of this compound can be achieved using reducing agents such as lithium aluminum hydride (LiAlH4) or sodium borohydride (NaBH4).
Substitution: The methoxy groups can be substituted with other functional groups through nucleophilic aromatic substitution reactions.
Common Reagents and Conditions:
Oxidation: Potassium permanganate (KMnO4) in an acidic medium.
Reduction: Lithium aluminum hydride (LiAlH4) in anhydrous ether.
Substitution: Sodium methoxide (NaOCH3) in methanol.
Major Products:
Oxidation: Quinones.
Reduction: Tetrahydroxybenzene.
Substitution: Various substituted benzene derivatives depending on the nucleophile used.
Mechanism of Action
The mechanism of action of 1,2,3,4-tetramethoxybenzene involves its interaction with various molecular targets and pathways. The methoxy groups on the benzene ring can participate in electron-donating interactions, making the compound a good electron donor. This property is exploited in reactions involving electron transfer, such as in the formation of molecular wires for electronic applications .
Comparison with Similar Compounds
1,2,3,4-Tetrahydroxybenzene: This compound is similar in structure but has hydroxyl groups instead of methoxy groups.
1,2,3,4-Tetramethylbenzene: This compound has methyl groups instead of methoxy groups.
1,2,3,5-Tetramethoxybenzene: This compound has methoxy groups at different positions on the benzene ring.
Uniqueness: 1,2,3,4-Tetramethoxybenzene is unique due to its specific arrangement of methoxy groups, which imparts distinct chemical and physical properties. The electron-donating nature of the methoxy groups enhances its reactivity in various chemical reactions, making it a valuable compound in synthetic chemistry .
Properties
IUPAC Name |
1,2,3,4-tetramethoxybenzene | |
---|---|---|
Source | PubChem | |
URL | https://pubchem.ncbi.nlm.nih.gov | |
Description | Data deposited in or computed by PubChem | |
InChI |
InChI=1S/C10H14O4/c1-11-7-5-6-8(12-2)10(14-4)9(7)13-3/h5-6H,1-4H3 | |
Source | PubChem | |
URL | https://pubchem.ncbi.nlm.nih.gov | |
Description | Data deposited in or computed by PubChem | |
InChI Key |
QCNHIJXDZKTWSA-UHFFFAOYSA-N | |
Source | PubChem | |
URL | https://pubchem.ncbi.nlm.nih.gov | |
Description | Data deposited in or computed by PubChem | |
Canonical SMILES |
COC1=C(C(=C(C=C1)OC)OC)OC | |
Source | PubChem | |
URL | https://pubchem.ncbi.nlm.nih.gov | |
Description | Data deposited in or computed by PubChem | |
Molecular Formula |
C10H14O4 | |
Source | PubChem | |
URL | https://pubchem.ncbi.nlm.nih.gov | |
Description | Data deposited in or computed by PubChem | |
DSSTOX Substance ID |
DTXSID50944046 | |
Record name | 1,2,3,4-Tetramethoxybenzene | |
Source | EPA DSSTox | |
URL | https://comptox.epa.gov/dashboard/DTXSID50944046 | |
Description | DSSTox provides a high quality public chemistry resource for supporting improved predictive toxicology. | |
Molecular Weight |
198.22 g/mol | |
Source | PubChem | |
URL | https://pubchem.ncbi.nlm.nih.gov | |
Description | Data deposited in or computed by PubChem | |
CAS No. |
21450-56-6 | |
Record name | 1,2,3,4-Tetramethoxybenzene | |
Source | EPA DSSTox | |
URL | https://comptox.epa.gov/dashboard/DTXSID50944046 | |
Description | DSSTox provides a high quality public chemistry resource for supporting improved predictive toxicology. | |
Synthesis routes and methods
Procedure details
Retrosynthesis Analysis
AI-Powered Synthesis Planning: Our tool employs the Template_relevance Pistachio, Template_relevance Bkms_metabolic, Template_relevance Pistachio_ringbreaker, Template_relevance Reaxys, Template_relevance Reaxys_biocatalysis model, leveraging a vast database of chemical reactions to predict feasible synthetic routes.
One-Step Synthesis Focus: Specifically designed for one-step synthesis, it provides concise and direct routes for your target compounds, streamlining the synthesis process.
Accurate Predictions: Utilizing the extensive PISTACHIO, BKMS_METABOLIC, PISTACHIO_RINGBREAKER, REAXYS, REAXYS_BIOCATALYSIS database, our tool offers high-accuracy predictions, reflecting the latest in chemical research and data.
Strategy Settings
Precursor scoring | Relevance Heuristic |
---|---|
Min. plausibility | 0.01 |
Model | Template_relevance |
Template Set | Pistachio/Bkms_metabolic/Pistachio_ringbreaker/Reaxys/Reaxys_biocatalysis |
Top-N result to add to graph | 6 |
Feasible Synthetic Routes
Q1: How does pile-fermentation affect the aroma profile of Pu-erh tea, specifically regarding 1,2,3,4-Tetramethoxybenzene?
A: Research indicates that this compound is a significant odorant in ripened Pu-erh tea (RIPT) and undergoes remarkable changes during the pile-fermentation process. [] This suggests that the fermentation process contributes to the formation and increase of this compound, leading to the characteristic aroma of RIPT, described as "stale" and "musty." [] In contrast, raw Pu-erh tea (RAPT), which doesn't undergo the same fermentation, exhibits a different aroma profile dominated by floral, sweet, and woody notes, primarily attributed to terpene alcohols, terpene ketones, and phenolic compounds. []
Disclaimer and Information on In-Vitro Research Products
Please be aware that all articles and product information presented on BenchChem are intended solely for informational purposes. The products available for purchase on BenchChem are specifically designed for in-vitro studies, which are conducted outside of living organisms. In-vitro studies, derived from the Latin term "in glass," involve experiments performed in controlled laboratory settings using cells or tissues. It is important to note that these products are not categorized as medicines or drugs, and they have not received approval from the FDA for the prevention, treatment, or cure of any medical condition, ailment, or disease. We must emphasize that any form of bodily introduction of these products into humans or animals is strictly prohibited by law. It is essential to adhere to these guidelines to ensure compliance with legal and ethical standards in research and experimentation.