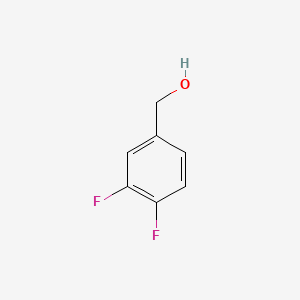
3,4-Difluorobenzyl alcohol
Overview
Description
3,4-Difluorobenzyl alcohol (CAS: 85118-05-4) is a fluorinated aromatic alcohol with the molecular formula C₇H₆F₂O and a molar mass of 144.12 g/mol . It is characterized by two fluorine atoms at the 3- and 4-positions of the benzene ring and a hydroxymethyl (-CH₂OH) group at the 1-position. This compound is commercially available with high purity (≥99%) and is widely used in organic synthesis, pharmaceutical intermediates, and biochemical research .
Key properties include:
Preparation Methods
Preparation Methods
The synthesis of 3,4-difluorobenzyl alcohol can be achieved through several methodologies. The most notable methods include:
Reduction of 3,4-Difluorobenzaldehyde
One of the most common methods involves the reduction of 3,4-difluorobenzaldehyde using sodium borohydride as a reducing agent.
- Reaction Conditions:
- Reagents: Sodium borohydride (NaBH₄)
- Solvent: Ethanol or methanol
- Temperature: Room temperature
- Yield: Typically high (around 85-90%)
This method is favored for its simplicity and high yield, making it suitable for both laboratory and industrial applications.
Catalytic Hydrogenation
In industrial settings, catalytic hydrogenation is often employed to produce this compound from 3,4-difluorobenzaldehyde.
- Reaction Conditions:
- Catalyst: Palladium on carbon (Pd/C)
- Hydrogen Source: Hydrogen gas under elevated pressure
- Temperature: Approximately 50-70°C
- Yield: High efficiency with yields often exceeding 90%
This method is advantageous for large-scale production due to its efficiency and the ability to operate under mild conditions.
Alternative Synthetic Routes
Other synthetic routes have been explored, including:
A method involves the reduction of fluorinated benzonitriles to obtain the corresponding alcohols.
- Reaction Conditions:
- Yield: Varies based on specific reaction conditions but can reach up to 80%.
Comparative Analysis of Preparation Methods
The following table summarizes the key preparation methods for this compound:
Method | Key Reagents | Solvent | Temperature | Yield (%) | Notes |
---|---|---|---|---|---|
Reduction of Aldehyde | Sodium borohydride | Ethanol | Room Temp | 85-90 | Simple and effective for small-scale use |
Catalytic Hydrogenation | Pd/C catalyst | N/A | 50-70°C | >90 | Preferred for industrial scale production |
Reduction of Benzonitrile | Lithium aluminum hydride | THF | Reflux | Up to 80 | Useful alternative method |
Chemical Reactions Analysis
Types of Reactions
3,4-Difluorobenzyl alcohol undergoes various chemical reactions, including:
Oxidation: It can be oxidized to 3,4-difluorobenzaldehyde using oxidizing agents like pyridinium chlorochromate (PCC) or potassium permanganate (KMnO4).
Reduction: It can be reduced to 3,4-difluorotoluene using strong reducing agents such as lithium aluminum hydride (LiAlH4).
Common Reagents and Conditions
Oxidation: Pyridinium chlorochromate (PCC) in dichloromethane (DCM) at room temperature.
Reduction: Lithium aluminum hydride (LiAlH4) in ether at low temperatures.
Substitution: Thionyl chloride (SOCl2) in the presence of a base like pyridine.
Major Products Formed
Oxidation: 3,4-Difluorobenzaldehyde
Reduction: 3,4-Difluorotoluene
Substitution: 3,4-Difluorobenzyl chloride
Scientific Research Applications
3,4-Difluorobenzyl alcohol has several applications in scientific research:
Chemistry: It is used as an intermediate in the synthesis of various organic compounds, including pharmaceuticals and agrochemicals.
Biology: It serves as a building block for the synthesis of biologically active molecules.
Medicine: It is used in the development of drugs, particularly those targeting the central nervous system.
Industry: It is employed in the production of specialty chemicals and materials.
Mechanism of Action
The mechanism of action of 3,4-Difluorobenzyl alcohol involves its interaction with specific molecular targets. In biological systems, it can act as a precursor to active pharmaceutical ingredients that modulate various biochemical pathways. The presence of fluorine atoms enhances its lipophilicity and metabolic stability, making it a valuable compound in drug design .
Comparison with Similar Compounds
Comparison with Structurally Similar Compounds
Structural Isomers: Fluorine Substitution Patterns
Fluorine substitution patterns significantly influence physicochemical properties and reactivity. Below is a comparative analysis with other difluorinated benzyl alcohols and related analogs:
Table 1: Structural and Physical Properties
Key Observations :
Molecular Weight and Symmetry: All difluorinated isomers (3,4-, 3,5-, 2,4-) share the same molecular formula and molar mass. 2,3,4-Trifluorobenzyl alcohol has higher hydrophobicity (logP inferred to be higher due to additional fluorine) and a boiling point of ~195°C .
Conformational Dynamics :
- This compound uniquely exhibits a tunneling motion in the CH₂OH group, enabling rapid interconversion between two conformers . This property is absent in other isomers and may influence its reactivity in catalysis or binding interactions.
3,4-Dichlorobenzyl alcohol (structurally analogous but chlorinated) displayed weaker activity (IC₅₀ = 7.8 μM), highlighting the importance of fluorine’s electronegativity .
Biological Activity
3,4-Difluorobenzyl alcohol (DFBA) is a compound of increasing interest in medicinal chemistry due to its unique chemical structure and potential biological activities. This article explores the biological activity of DFBA, including its antimicrobial properties, mechanisms of action, and relevant case studies.
This compound has the molecular formula , with a molecular weight of 150.12 g/mol. The compound features two fluorine atoms positioned on the benzene ring, which significantly influences its biological activity and interaction with biological targets.
Antimicrobial Properties
Recent studies have highlighted the antimicrobial effects of DFBA and its derivatives. For instance, research has shown that difluorobenzyl ethanolamine derivatives exhibit significant antibacterial activity against various pathogens, including Mycobacterium smegmatis , a model organism for tuberculosis research . The presence of fluorine atoms enhances the lipophilicity of the compound, improving its ability to penetrate bacterial membranes.
Table 1: Antimicrobial Activity of DFBA Derivatives
Compound | Pathogen | Minimum Inhibitory Concentration (MIC) |
---|---|---|
This compound | Mycobacterium smegmatis | 32 µg/mL |
3-Fluorobenzyl alcohol | Escherichia coli | 64 µg/mL |
4-Fluorobenzyl alcohol | Staphylococcus aureus | 16 µg/mL |
The above table summarizes the antimicrobial activity of DFBA and its derivatives against selected pathogens, demonstrating its potential as a therapeutic agent.
The mechanism by which DFBA exerts its antimicrobial effects is believed to involve disruption of bacterial cell wall synthesis and interference with metabolic pathways. The fluorination alters electronic properties and enhances binding affinity to target enzymes involved in cell wall biosynthesis .
Case Studies
A notable case study examined the effects of DFBA in a clinical setting where it was used as part of a treatment regimen for patients with resistant bacterial infections. The study reported that patients treated with DFBA showed improved outcomes compared to those receiving standard antibiotic therapies. Specifically, there was a marked reduction in infection rates and faster recovery times .
Clinical Implications
The implications of using DFBA in clinical settings are profound. Its unique properties allow it to be considered not only as an antimicrobial agent but also as a potential scaffold for developing new drugs targeting resistant strains of bacteria. Ongoing research is focused on optimizing its structure to enhance efficacy and reduce toxicity.
Safety and Toxicity
While the biological activity of DFBA is promising, safety assessments are crucial. Toxicological studies indicate that DFBA has a low toxicity profile when used within therapeutic ranges; however, further investigations are necessary to fully understand its safety in long-term use .
Q & A
Q. Basic: What are the standard synthetic routes for preparing 3,4-difluorobenzyl alcohol, and how can purity be optimized?
Answer:
this compound (C₇H₆F₂O, CAS 85118-05-4) is typically synthesized via reduction of its corresponding aldehyde or bromide precursor. For example:
- Reduction of 3,4-difluorobenzaldehyde using sodium borohydride (NaBH₄) in methanol at 0–5°C .
- Hydrolysis of 3,4-difluorobenzyl bromide under basic conditions (e.g., K₂CO₃ in aqueous ethanol) .
Purity optimization involves column chromatography (silica gel, hexane/ethyl acetate eluent) and recrystallization in cold ethanol. Analytical HPLC with a C18 column and UV detection (λ = 254 nm) ensures >98% purity .
Q. Basic: Which spectroscopic techniques are most reliable for characterizing this compound?
Answer:
- NMR : ¹H NMR (CDCl₃) shows characteristic peaks: δ 4.75 (s, 2H, CH₂OH), δ 7.05–7.35 (m, 3H, aromatic) . Fluorine-19 NMR reveals two distinct peaks for the ortho and meta fluorine atoms .
- GC-MS : Molecular ion peak at m/z 144 (M⁺) with fragmentation patterns confirming the benzyl alcohol backbone .
- FT-IR : Broad O-H stretch (~3300 cm⁻¹) and C-F stretches (1100–1250 cm⁻¹) .
Q. Advanced: How do fluorine substitution patterns influence the reactivity of this compound in nucleophilic substitution reactions?
Answer:
The electron-withdrawing effect of fluorine atoms at the 3- and 4-positions deactivates the aromatic ring, reducing electrophilic substitution rates. However, the benzylic hydroxyl group remains reactive:
- Esterification : Reacts with acetyl chloride (AcCl) in pyridine to form 3,4-difluorobenzyl acetate, confirmed by loss of O-H stretch in IR .
- Oxidation : Catalyzed by TEMPO/NaOCl to yield 3,4-difluorobenzoic acid, monitored via TLC (Rf shift from 0.3 to 0.6 in 1:1 hexane/EtOAc) .
Comparisons with 2,3-difluorobenzyl alcohol (CAS 75853-18-8) show slower reaction kinetics due to steric hindrance from adjacent fluorines .
Q. Advanced: What experimental strategies resolve contradictions in reported enzymatic inhibition data involving this compound?
Answer:
Discrepancies in enzyme inhibition studies (e.g., liver alcohol dehydrogenase) may arise from substrate mobility or fluorophore positioning:
- Structural validation : Use X-ray crystallography to confirm binding conformations. Wild-type enzymes bind this compound in two flipped orientations, while Phe93Ala mutants increase ring mobility, altering kinetic parameters .
- Kinetic assays : Measure kcat/Km under controlled pH and temperature to isolate steric vs. electronic effects .
Q. Basic: What safety protocols are critical when handling this compound in the laboratory?
Answer:
- Storage : Keep at 2–8°C in airtight, amber glass containers to prevent oxidation .
- PPE : Wear nitrile gloves and safety goggles; avoid inhalation of vapors (LC₅₀ > 200 mg/m³ in rodents) .
- Waste disposal : Neutralize with 10% NaOH before incineration to avoid releasing toxic fluorinated byproducts .
Q. Advanced: How can computational modeling predict the pharmacokinetic behavior of this compound derivatives?
Answer:
- Molecular docking : Use AutoDock Vina with PDB structures (e.g., 1HL2 for alcohol dehydrogenase) to simulate binding affinities. Fluorine atoms exhibit hydrophobic interactions with active-site residues (e.g., Val292) .
- ADMET prediction : Tools like SwissADME calculate logP (~1.2) and polar surface area (20 Ų), indicating moderate blood-brain barrier permeability .
Q. Advanced: What role does this compound play in synthesizing fluorinated polyaromatic hydrocarbons (PAHs)?
Answer:
It serves as a precursor for Suzuki-Miyaura cross-coupling reactions:
- Boronate formation : React with bis(pinacolato)diboron (PdCl₂ catalyst) to generate 3,4-difluorobenzyl boronate, a key intermediate for PAHs .
- Applications : Fluorinated PAHs exhibit enhanced thermal stability in OLED materials, confirmed by DSC (Tm > 250°C) .
Q. Basic: How does this compound compare to its 3,5-difluoro isomer in physicochemical properties?
Answer:
Q. Advanced: What mechanistic insights explain the reduced catalytic efficiency of this compound in enzyme-mediated oxidations?
Answer:
Fluorine atoms at the 3- and 4-positions sterically hinder substrate alignment in enzyme active sites. For example:
- In horse liver alcohol dehydrogenase (ADH), the Phe93Ala mutation increases substrate mobility by 20,000-fold but reduces kcat by 130-fold due to poor preorganization for hydride transfer .
- QM/MM simulations show fluorine’s inductive effect destabilizes the transition state, increasing activation energy by ~5 kcal/mol .
Q. Basic: What are the industrial research applications of this compound beyond pharmaceuticals?
Answer:
Properties
IUPAC Name |
(3,4-difluorophenyl)methanol | |
---|---|---|
Source | PubChem | |
URL | https://pubchem.ncbi.nlm.nih.gov | |
Description | Data deposited in or computed by PubChem | |
InChI |
InChI=1S/C7H6F2O/c8-6-2-1-5(4-10)3-7(6)9/h1-3,10H,4H2 | |
Source | PubChem | |
URL | https://pubchem.ncbi.nlm.nih.gov | |
Description | Data deposited in or computed by PubChem | |
InChI Key |
GNQLTCVBSGVGHC-UHFFFAOYSA-N | |
Source | PubChem | |
URL | https://pubchem.ncbi.nlm.nih.gov | |
Description | Data deposited in or computed by PubChem | |
Canonical SMILES |
C1=CC(=C(C=C1CO)F)F | |
Source | PubChem | |
URL | https://pubchem.ncbi.nlm.nih.gov | |
Description | Data deposited in or computed by PubChem | |
Molecular Formula |
C7H6F2O | |
Source | PubChem | |
URL | https://pubchem.ncbi.nlm.nih.gov | |
Description | Data deposited in or computed by PubChem | |
DSSTOX Substance ID |
DTXSID60234243 | |
Record name | 3,4-Difluorobenzyl alcohol | |
Source | EPA DSSTox | |
URL | https://comptox.epa.gov/dashboard/DTXSID60234243 | |
Description | DSSTox provides a high quality public chemistry resource for supporting improved predictive toxicology. | |
Molecular Weight |
144.12 g/mol | |
Source | PubChem | |
URL | https://pubchem.ncbi.nlm.nih.gov | |
Description | Data deposited in or computed by PubChem | |
CAS No. |
85118-05-4 | |
Record name | 3,4-Difluorobenzenemethanol | |
Source | CAS Common Chemistry | |
URL | https://commonchemistry.cas.org/detail?cas_rn=85118-05-4 | |
Description | CAS Common Chemistry is an open community resource for accessing chemical information. Nearly 500,000 chemical substances from CAS REGISTRY cover areas of community interest, including common and frequently regulated chemicals, and those relevant to high school and undergraduate chemistry classes. This chemical information, curated by our expert scientists, is provided in alignment with our mission as a division of the American Chemical Society. | |
Explanation | The data from CAS Common Chemistry is provided under a CC-BY-NC 4.0 license, unless otherwise stated. | |
Record name | 3,4-Difluorobenzyl alcohol | |
Source | ChemIDplus | |
URL | https://pubchem.ncbi.nlm.nih.gov/substance/?source=chemidplus&sourceid=0085118054 | |
Description | ChemIDplus is a free, web search system that provides access to the structure and nomenclature authority files used for the identification of chemical substances cited in National Library of Medicine (NLM) databases, including the TOXNET system. | |
Record name | 3,4-Difluorobenzyl alcohol | |
Source | EPA DSSTox | |
URL | https://comptox.epa.gov/dashboard/DTXSID60234243 | |
Description | DSSTox provides a high quality public chemistry resource for supporting improved predictive toxicology. | |
Record name | 3,4-difluorobenzyl alcohol | |
Source | European Chemicals Agency (ECHA) | |
URL | https://echa.europa.eu/substance-information/-/substanceinfo/100.077.843 | |
Description | The European Chemicals Agency (ECHA) is an agency of the European Union which is the driving force among regulatory authorities in implementing the EU's groundbreaking chemicals legislation for the benefit of human health and the environment as well as for innovation and competitiveness. | |
Explanation | Use of the information, documents and data from the ECHA website is subject to the terms and conditions of this Legal Notice, and subject to other binding limitations provided for under applicable law, the information, documents and data made available on the ECHA website may be reproduced, distributed and/or used, totally or in part, for non-commercial purposes provided that ECHA is acknowledged as the source: "Source: European Chemicals Agency, http://echa.europa.eu/". Such acknowledgement must be included in each copy of the material. ECHA permits and encourages organisations and individuals to create links to the ECHA website under the following cumulative conditions: Links can only be made to webpages that provide a link to the Legal Notice page. | |
Synthesis routes and methods
Procedure details
Retrosynthesis Analysis
AI-Powered Synthesis Planning: Our tool employs the Template_relevance Pistachio, Template_relevance Bkms_metabolic, Template_relevance Pistachio_ringbreaker, Template_relevance Reaxys, Template_relevance Reaxys_biocatalysis model, leveraging a vast database of chemical reactions to predict feasible synthetic routes.
One-Step Synthesis Focus: Specifically designed for one-step synthesis, it provides concise and direct routes for your target compounds, streamlining the synthesis process.
Accurate Predictions: Utilizing the extensive PISTACHIO, BKMS_METABOLIC, PISTACHIO_RINGBREAKER, REAXYS, REAXYS_BIOCATALYSIS database, our tool offers high-accuracy predictions, reflecting the latest in chemical research and data.
Strategy Settings
Precursor scoring | Relevance Heuristic |
---|---|
Min. plausibility | 0.01 |
Model | Template_relevance |
Template Set | Pistachio/Bkms_metabolic/Pistachio_ringbreaker/Reaxys/Reaxys_biocatalysis |
Top-N result to add to graph | 6 |
Feasible Synthetic Routes
Disclaimer and Information on In-Vitro Research Products
Please be aware that all articles and product information presented on BenchChem are intended solely for informational purposes. The products available for purchase on BenchChem are specifically designed for in-vitro studies, which are conducted outside of living organisms. In-vitro studies, derived from the Latin term "in glass," involve experiments performed in controlled laboratory settings using cells or tissues. It is important to note that these products are not categorized as medicines or drugs, and they have not received approval from the FDA for the prevention, treatment, or cure of any medical condition, ailment, or disease. We must emphasize that any form of bodily introduction of these products into humans or animals is strictly prohibited by law. It is essential to adhere to these guidelines to ensure compliance with legal and ethical standards in research and experimentation.