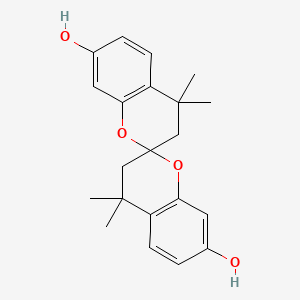
Spirobicromane
Overview
Description
Spirobicromane (C₂₄H₂₆O₄) is a synthetic compound identified for its potent antagonistic activity against estrogen receptor alpha (ERα), a key target in hormone-dependent cancers and endocrine therapies. Structurally, it features a spirocyclic core with two chromane moieties linked via a sp³-hybridized carbon atom (Figure 1). This unique architecture distinguishes it from conventional ERα ligands like 17β-estradiol (E2) or selective estrogen receptor modulators (SERMs) such as tamoxifen.
This compound demonstrates dose-dependent inhibition of ERα-mediated transcriptional activity, achieving up to 90% suppression of 1 nM E2-induced agonism at 10 μM concentration. Its mechanism involves competitive binding to ERα’s ligand-binding domain (LBD), inducing conformational changes that disrupt co-activator recruitment.
Preparation Methods
Condensation Reactions
One of the most common methods for synthesizing spirobicromane involves a condensation reaction between specific aromatic aldehydes and ketones.
Reagents :
- 4-Methyl-2-hydroxybenzaldehyde
- 4-Methyl-2-hydroxyacetophenone
- Sodium hydroxide (as a base)
-
- The reaction typically proceeds under reflux conditions, where the reactants are heated together in the presence of sodium hydroxide to facilitate the condensation process.
-
- This method has been reported to yield this compound with satisfactory purity levels, often requiring subsequent purification steps such as recrystallization or chromatography to achieve desired specifications.
One-Pot Three-Component Reactions
A more recent approach involves a one-pot three-component reaction that simplifies the synthesis process.
Reagents :
- Ninhydrin or isatin
- Malononitrile
- Beta-dicarbonyl compounds
- 4-Dimethylaminopyridine (as a catalyst)
-
- A mixture of ninhydrin (or isatin) and malononitrile is stirred at room temperature, followed by the addition of beta-dicarbonyl compounds. The reaction is monitored using thin-layer chromatography (TLC) until completion.
Results :
Industrial Production Techniques
In industrial settings, optimized production methods are crucial for scaling up the synthesis of this compound.
-
- Reaction temperature, time, and concentration of reagents are meticulously controlled to maximize yield and purity.
-
- Following synthesis, products are typically purified through recrystallization or chromatography to ensure high-quality output suitable for commercial applications.
Summary Table of Preparation Methods
Method | Key Reagents | Conditions | Yield (%) | Purity (%) |
---|---|---|---|---|
Condensation Reaction | 4-Methyl-2-hydroxybenzaldehyde, sodium hydroxide | Reflux | Varies | Varies |
One-Pot Three-Component Reaction | Ninhydrin, malononitrile, beta-dicarbonyl compounds | Room temperature | >80 | High |
Industrial Production | Optimized reagents and conditions | Controlled environment | High | >95 |
Chemical Reactions Analysis
Types of Reactions: Spirobicromane undergoes various chemical reactions, including:
Oxidation: It can be oxidized using reagents such as potassium permanganate or chromium trioxide, leading to the formation of quinone derivatives.
Reduction: Reduction reactions can be carried out using reducing agents like sodium borohydride or lithium aluminum hydride, resulting in the formation of dihydro derivatives.
Common Reagents and Conditions:
Oxidation: Potassium permanganate in an acidic medium.
Reduction: Sodium borohydride in methanol.
Substitution: Chlorine or bromine in the presence of a Lewis acid catalyst.
Major Products Formed:
Oxidation: Quinone derivatives.
Reduction: Dihydro derivatives.
Substitution: Halogenated this compound derivatives.
Scientific Research Applications
Medicinal Chemistry
2.1 Anticancer Activity
Recent studies have investigated the anticancer properties of spirobicromane derivatives. For instance, a synthesized copolyester containing this compound was evaluated for its in vitro anticancer activity against lung cancer cell lines (Hep-2). The results indicated significant cytotoxic effects, suggesting that this compound derivatives may serve as potential anticancer agents.
Concentration (μg/mL) | Cell Viability (%) |
---|---|
1000 | 13.46 |
500 | 26.92 |
250 | 38.46 |
125 | 51.92 |
Control | 100 |
The above table summarizes the cell viability results from the MTT assay, demonstrating the effectiveness of this compound derivatives in inhibiting cancer cell growth .
2.2 Antimicrobial Properties
This compound has also been studied for its antimicrobial properties. Research indicates that certain derivatives exhibit strong antibacterial activity against both Gram-positive and Gram-negative bacteria, making them suitable candidates for developing new antimicrobial agents .
Material Science Applications
3.1 Polymer Chemistry
In polymer chemistry, this compound serves as a building block for synthesizing advanced materials. Its unique structure allows for the design of polymers with specific mechanical and thermal properties, which can be utilized in various industrial applications.
3.2 Nanotechnology
The integration of this compound into nanotechnology has been explored, particularly in the development of phenolic-enabled nanomaterials. These materials leverage the chemical properties of this compound to create nanoparticles with enhanced biocompatibility and reactivity, suitable for biomedical applications such as drug delivery systems .
Case Studies
4.1 Study on Anticancer Activity
A notable case study involved synthesizing a series of this compound derivatives and evaluating their effects on cancer cell lines. The study found that specific modifications to the this compound structure significantly enhanced its cytotoxicity against cancer cells, indicating a promising avenue for drug development .
4.2 Antimicrobial Efficacy Assessment
Another study assessed the antimicrobial efficacy of this compound derivatives against common pathogens. The findings revealed that certain derivatives exhibited potent inhibitory effects on bacterial growth, suggesting their potential use in developing new antimicrobial therapies .
Mechanism of Action
The mechanism of action of spirobicromane involves its interaction with various molecular targets. Its phenolic hydroxyl groups can participate in hydrogen bonding and redox reactions, which contribute to its antioxidant properties. Additionally, its spiro structure allows for unique interactions with biological molecules, potentially influencing cellular pathways involved in inflammation and cancer.
Comparison with Similar Compounds
Comparison with Structurally and Functionally Similar Compounds
Spirobicromane belongs to a class of ERα antagonists characterized by tricyclic bisphenol derivatives. Below, we compare its structural, biochemical, and functional properties with four analogs: BPM, BPP, α,α,α′-Tris(4-hydroxyphenyl)-1-ethyl-4-isopropylbenzene, and α,α′-Bis(4-hydroxy-3,5-dimethylphenyl)-1,4-diisopropylbenzene.
Table 1: Structural and Functional Comparison of this compound and Analogs
Key Findings:
Structural Divergence: this compound’s spirocyclic chromane system introduces steric constraints absent in linear or branched tricyclic analogs. This rigidity may explain its preferential binding to ERα’s non-active conformation (PDB: 3UUC), contrasting with BPM/BPP analogs that stabilize the active conformation (PDB: 3UUA). Unlike other analogs, this compound lacks the conjugated tricyclic bisphenol motif, reducing π-π stacking interactions but enhancing metabolic stability.
Functional Overlaps :
- All compounds exhibit equivalent ERα antagonistic potency (90% inhibition at 10 μM), suggesting that divergent structures converge on a common inhibitory pathway.
- Hydrophobic substituents (e.g., isopropyl or methyl groups) correlate with improved binding affinity across analogs, as seen in BPP and α,α′-Bis(4-hydroxy-3,5-dimethylphenyl)-....
Mechanistic Insights: Molecular docking studies reveal that this compound’s chromane rings occupy ERα’s helix 12 (H12) pocket, preventing H12 repositioning required for co-activator binding. In contrast, BPM and BPP analogs engage Asp351 and Glu353 residues via hydrogen bonding, a feature less prominent in this compound due to its non-planar structure.
Implications for Drug Design
This compound’s unique spirocyclic scaffold provides a privileged structure for developing ERα antagonists with minimized off-target effects. Its structural dissimilarity from endogenous ligands (e.g., E2) and SERMs reduces the risk of cross-reactivity with ERβ or other nuclear receptors. However, its synthetic complexity and bioavailability relative to simpler tricyclic analogs (e.g., BPM) warrant further optimization.
Biological Activity
Spirobicromane is a compound of interest due to its potential biological activities, particularly in relation to estrogen receptor modulation. This article reviews the biological activity of this compound, focusing on its mechanisms of action, pharmacological implications, and relevant case studies.
Chemical Structure and Properties
This compound is characterized by a unique spiro compound structure that includes a bicyclic framework. Its structural configuration allows for various interactions with biological targets, particularly hormone receptors.
Recent studies have indicated that this compound exhibits antagonistic activity against estrogen receptors (ER), specifically ERα. In competitive binding assays, this compound demonstrated the ability to inhibit the agonistic activity of estradiol (E2) in a dose-dependent manner. At concentrations up to 10 µM, it reduced E2-mediated ERα activity by approximately 90% . This suggests that this compound may disrupt the active conformation of ERα, preventing the recruitment of coactivators necessary for transcriptional activation.
Biological Activity Overview
The biological activities of this compound can be summarized as follows:
Case Studies and Research Findings
-
Estrogen Receptor Binding Studies
- A study evaluated the binding affinity of this compound alongside other bisphenol derivatives. The results showed that while several compounds exhibited strong binding affinities for ERα, this compound's unique structure allowed it to function effectively as an antagonist by preventing the necessary conformational changes in the receptor .
- Cocrystal Formation
- Antiproliferative Activity
Q & A
Q. Basic: How can researchers systematically identify gaps in existing literature on Spirobicromane?
Methodological Answer: Begin with a structured literature review using databases like PubMed, Scopus, and Web of Science. Apply search terms such as "this compound pharmacokinetics," "this compound synthesis pathways," or "this compound in vitro activity." Use Boolean operators (AND/OR/NOT) to refine results. Critically analyze review articles and original studies to identify inconsistencies, understudied mechanisms (e.g., metabolic pathways), or conflicting results. Tools like PICO (Population, Intervention, Comparison, Outcome) or SPIDER (Sample, Phenomenon of Interest, Design, Evaluation, Research Type) can frame research questions around these gaps. For example, "What are the comparative efficacy outcomes (O) of this compound (I) versus standard analogs (C) in in vitro models (P)?"
Q. Basic: What experimental design principles should guide initial in vitro studies of this compound?
Methodological Answer:
Adopt a hypothesis-driven approach with clear variables (e.g., concentration, exposure time, cell lines). Include positive/negative controls (e.g., known inhibitors or vehicle solutions) to validate assay conditions. Use dose-response curves to establish IC50 values and assess toxicity thresholds. Ensure reproducibility by triplicate experiments and statistical power analysis to determine sample size . Document protocols in alignment with FAIR principles (Findable, Accessible, Interoperable, Reusable) . For example, in cytotoxicity assays, report cell viability using standardized metrics (e.g., % inhibition ± SEM) and reference established guidelines like OECD TG 129 for in vitro systems.
Q. Advanced: How can researchers resolve contradictions in reported data on this compound’s mechanism of action?
Methodological Answer:
Contradictions may arise from methodological variability (e.g., assay conditions, cell models). Conduct a meta-analysis of published data to identify confounding factors, such as differences in solvent polarity (DMSO vs. ethanol) or incubation times. Validate findings using orthogonal techniques:
- Compare enzyme inhibition assays (e.g., fluorometric vs. colorimetric).
- Use CRISPR-edited cell lines to isolate target pathways.
- Apply advanced analytics like molecular docking to predict binding affinities .
Address discrepancies through collaborative replication studies and transparent reporting of raw data .
Q. Advanced: What strategies optimize the synthesis protocol of this compound for reproducibility in academic labs?
Methodological Answer:
Standardize synthetic steps using reaction calorimetry to monitor exothermic events and reduce batch variability. Characterize intermediates via HPLC-MS and NMR (1H, 13C) at each stage to confirm purity (>95%). Document solvent gradients, catalyst loadings, and temperature profiles in detail. For chiral centers, specify enantiomeric excess using chiral stationary-phase chromatography . Share protocols via platforms like protocols.io to facilitate peer validation .
Q. Basic: What analytical techniques are critical for characterizing this compound’s physicochemical properties?
Methodological Answer:
- Purity: HPLC with UV/Vis or ELSD detection (USP/EP guidelines).
- Stability: Forced degradation studies under thermal, photolytic, and hydrolytic stress (ICH Q1A).
- Solubility: Shake-flask method with biorelevant media (FaSSIF/FeSSIF).
- LogP: Octanol-water partition coefficient via shake-flask or chromatographic derivation .
Report data in tables with descriptive titles, footnotes, and units (e.g., "Table 1: Stability profile of this compound under accelerated conditions") .
Q. Advanced: How should researchers design translational studies to evaluate this compound’s in vivo efficacy?
Methodological Answer:
Align with ARRIVE 2.0 guidelines for preclinical studies. Select animal models based on translational relevance (e.g., PDX models for oncology). Include pharmacokinetic endpoints (Cmax, AUC, t1/2) and biomarker validation (e.g., Western blot for target engagement). Use randomized block designs to control for inter-individual variability and blinded outcome assessments . For dose extrapolation, apply allometric scaling or physiologically based pharmacokinetic (PBPK) modeling .
Q. Basic: What statistical methods are appropriate for analyzing this compound’s dose-response data?
Methodological Answer:
Use nonlinear regression (e.g., four-parameter logistic model) to calculate EC50/IC50 values. Validate assumptions of normality (Shapiro-Wilk test) and homogeneity of variance (Levene’s test). For multi-group comparisons, apply ANOVA with post-hoc corrections (Tukey’s HSD). Report effect sizes (Cohen’s d) and 95% confidence intervals . Avoid overstating "significance"; instead, emphasize biological relevance .
Q. Advanced: How can researchers integrate omics data to elucidate this compound’s polypharmacology?
Methodological Answer:
Combine transcriptomics (RNA-seq), proteomics (LC-MS/MS), and metabolomics (NMR) datasets. Use pathway enrichment tools (DAVID, MetaboAnalyst) to identify perturbed networks (e.g., apoptosis, oxidative stress). Validate hypotheses with CRISPR interference (CRISPRi) or small-molecule probes. Employ machine learning (e.g., random forest) to predict off-target interactions . Share data in public repositories (GEO, PRIDE) to enhance reproducibility .
Q. Basic: What ethical considerations apply to studies involving this compound in animal models?
Methodological Answer:
Follow institutional Animal Care and Use Committee (IACUC) protocols. Justify species selection and sample size using the "3Rs" (Replacement, Reduction, Refinement). Monitor welfare indicators (e.g., body weight, behavior) and establish humane endpoints. Disclose conflicts of interest and funding sources in publications .
Q. Advanced: How can researchers address batch-to-batch variability in this compound for long-term studies?
Methodological Answer:
Implement Quality by Design (QbD) principles:
- Define Critical Quality Attributes (CQAs): Purity, crystallinity, particle size.
- Use Design of Experiments (DoE) to optimize synthesis parameters.
- Apply PAT (Process Analytical Technology) for real-time monitoring.
Archive reference standards under controlled conditions (-20°C, desiccated) and validate stability every 6 months .
Properties
IUPAC Name |
4,4,4',4'-tetramethyl-2,2'-spirobi[3H-chromene]-7,7'-diol | |
---|---|---|
Source | PubChem | |
URL | https://pubchem.ncbi.nlm.nih.gov | |
Description | Data deposited in or computed by PubChem | |
InChI |
InChI=1S/C21H24O4/c1-19(2)11-21(24-17-9-13(22)5-7-15(17)19)12-20(3,4)16-8-6-14(23)10-18(16)25-21/h5-10,22-23H,11-12H2,1-4H3 | |
Source | PubChem | |
URL | https://pubchem.ncbi.nlm.nih.gov | |
Description | Data deposited in or computed by PubChem | |
InChI Key |
BWQOXWHQZXVXSD-UHFFFAOYSA-N | |
Source | PubChem | |
URL | https://pubchem.ncbi.nlm.nih.gov | |
Description | Data deposited in or computed by PubChem | |
Canonical SMILES |
CC1(CC2(CC(C3=C(O2)C=C(C=C3)O)(C)C)OC4=C1C=CC(=C4)O)C | |
Source | PubChem | |
URL | https://pubchem.ncbi.nlm.nih.gov | |
Description | Data deposited in or computed by PubChem | |
Molecular Formula |
C21H24O4 | |
Source | PubChem | |
URL | https://pubchem.ncbi.nlm.nih.gov | |
Description | Data deposited in or computed by PubChem | |
DSSTOX Substance ID |
DTXSID10343778 | |
Record name | 4,4,4',4'-tetramethyl-2,2'-spirobi[3H-chromene]-7,7'-diol | |
Source | EPA DSSTox | |
URL | https://comptox.epa.gov/dashboard/DTXSID10343778 | |
Description | DSSTox provides a high quality public chemistry resource for supporting improved predictive toxicology. | |
Molecular Weight |
340.4 g/mol | |
Source | PubChem | |
URL | https://pubchem.ncbi.nlm.nih.gov | |
Description | Data deposited in or computed by PubChem | |
CAS No. |
3127-14-8 | |
Record name | 4,4,4',4'-tetramethyl-2,2'-spirobi[3H-chromene]-7,7'-diol | |
Source | EPA DSSTox | |
URL | https://comptox.epa.gov/dashboard/DTXSID10343778 | |
Description | DSSTox provides a high quality public chemistry resource for supporting improved predictive toxicology. | |
Record name | Spirobicromane | |
Source | European Chemicals Agency (ECHA) | |
URL | https://echa.europa.eu/information-on-chemicals | |
Description | The European Chemicals Agency (ECHA) is an agency of the European Union which is the driving force among regulatory authorities in implementing the EU's groundbreaking chemicals legislation for the benefit of human health and the environment as well as for innovation and competitiveness. | |
Explanation | Use of the information, documents and data from the ECHA website is subject to the terms and conditions of this Legal Notice, and subject to other binding limitations provided for under applicable law, the information, documents and data made available on the ECHA website may be reproduced, distributed and/or used, totally or in part, for non-commercial purposes provided that ECHA is acknowledged as the source: "Source: European Chemicals Agency, http://echa.europa.eu/". Such acknowledgement must be included in each copy of the material. ECHA permits and encourages organisations and individuals to create links to the ECHA website under the following cumulative conditions: Links can only be made to webpages that provide a link to the Legal Notice page. | |
Retrosynthesis Analysis
AI-Powered Synthesis Planning: Our tool employs the Template_relevance Pistachio, Template_relevance Bkms_metabolic, Template_relevance Pistachio_ringbreaker, Template_relevance Reaxys, Template_relevance Reaxys_biocatalysis model, leveraging a vast database of chemical reactions to predict feasible synthetic routes.
One-Step Synthesis Focus: Specifically designed for one-step synthesis, it provides concise and direct routes for your target compounds, streamlining the synthesis process.
Accurate Predictions: Utilizing the extensive PISTACHIO, BKMS_METABOLIC, PISTACHIO_RINGBREAKER, REAXYS, REAXYS_BIOCATALYSIS database, our tool offers high-accuracy predictions, reflecting the latest in chemical research and data.
Strategy Settings
Precursor scoring | Relevance Heuristic |
---|---|
Min. plausibility | 0.01 |
Model | Template_relevance |
Template Set | Pistachio/Bkms_metabolic/Pistachio_ringbreaker/Reaxys/Reaxys_biocatalysis |
Top-N result to add to graph | 6 |
Feasible Synthetic Routes
Disclaimer and Information on In-Vitro Research Products
Please be aware that all articles and product information presented on BenchChem are intended solely for informational purposes. The products available for purchase on BenchChem are specifically designed for in-vitro studies, which are conducted outside of living organisms. In-vitro studies, derived from the Latin term "in glass," involve experiments performed in controlled laboratory settings using cells or tissues. It is important to note that these products are not categorized as medicines or drugs, and they have not received approval from the FDA for the prevention, treatment, or cure of any medical condition, ailment, or disease. We must emphasize that any form of bodily introduction of these products into humans or animals is strictly prohibited by law. It is essential to adhere to these guidelines to ensure compliance with legal and ethical standards in research and experimentation.