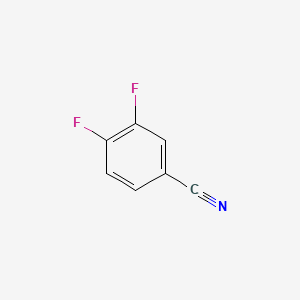
3,4-Difluorobenzonitrile
Overview
Description
3,4-Difluorobenzonitrile is an organic compound with the molecular formula C7H3F2N. It is a derivative of benzonitrile, where two hydrogen atoms on the benzene ring are replaced by fluorine atoms at the 3 and 4 positions. This compound is a white to pale cream crystalline solid with a melting point of 52-54°C .
Preparation Methods
Synthetic Routes and Reaction Conditions: One common method for synthesizing 3,4-Difluorobenzonitrile involves the reaction of 3,4-dichlorobenzonitrile with potassium fluoride in the presence of a phase transfer catalyst such as bis-(N,N’-1,3-dimethyl-2-imidazolinyl)-ammonium chloride. The reaction is carried out at a lower temperature with a short reaction time, yielding a high purity product .
Another method involves the reaction of 1,2-difluorobenzene with trichloroacetic chloride in the presence of a Lewis acid catalyst to form 3,4-difluoro-(alpha, alpha, alpha-trichloroacetic) benzene. This intermediate is then reacted with ammonia to obtain 3,4-difluorobenzamide, which is further dehydrated using a halogen-containing dehydration reagent and a catalyst to produce this compound .
Industrial Production Methods: The industrial production of this compound typically follows the synthetic routes mentioned above, with optimizations for large-scale production. The use of recyclable solvents and catalysts, as well as the recrystallization of by-products, helps in reducing production costs and environmental impact .
Chemical Reactions Analysis
Types of Reactions: 3,4-Difluorobenzonitrile undergoes various chemical reactions, including:
Substitution Reactions: It can participate in nucleophilic aromatic substitution reactions due to the presence of electron-withdrawing fluorine atoms.
Reduction Reactions: The nitrile group can be reduced to form corresponding amines.
Oxidation Reactions: The compound can be oxidized to form carboxylic acids.
Common Reagents and Conditions:
Substitution Reactions: Common reagents include nucleophiles such as amines or thiols, often under basic conditions.
Reduction Reactions: Catalytic hydrogenation or the use of reducing agents like lithium aluminum hydride.
Oxidation Reactions: Strong oxidizing agents such as potassium permanganate or chromium trioxide.
Major Products Formed:
Substitution Reactions: Substituted benzonitriles.
Reduction Reactions: 3,4-Difluoroaniline.
Oxidation Reactions: 3,4-Difluorobenzoic acid
Scientific Research Applications
3,4-Difluorobenzonitrile is used in various scientific research applications, including:
Chemistry: As a building block for the synthesis of more complex fluorinated organic compounds.
Biology: In the development of fluorinated pharmaceuticals and agrochemicals.
Medicine: As an intermediate in the synthesis of potential drug candidates.
Industry: In the production of fluorophenoxy herbicides and fluorine-substituted benzyl amides
Mechanism of Action
The mechanism of action of 3,4-Difluorobenzonitrile depends on its application. In the context of herbicides, it acts by inhibiting specific enzymes in the target plants, leading to their death. The fluorine atoms enhance the compound’s ability to interact with biological targets, increasing its efficacy .
Comparison with Similar Compounds
- 2,3-Difluorobenzonitrile
- 2,4-Difluorobenzonitrile
- 3,5-Difluorobenzonitrile
- 3,4-Dichlorobenzonitrile
- 2-Fluorobenzonitrile
Comparison: 3,4-Difluorobenzonitrile is unique due to the specific positioning of the fluorine atoms, which influences its reactivity and interaction with other molecules. Compared to its isomers, it may exhibit different physical properties, such as melting point and solubility, and varying reactivity in chemical reactions .
Properties
IUPAC Name |
3,4-difluorobenzonitrile | |
---|---|---|
Source | PubChem | |
URL | https://pubchem.ncbi.nlm.nih.gov | |
Description | Data deposited in or computed by PubChem | |
InChI |
InChI=1S/C7H3F2N/c8-6-2-1-5(4-10)3-7(6)9/h1-3H | |
Source | PubChem | |
URL | https://pubchem.ncbi.nlm.nih.gov | |
Description | Data deposited in or computed by PubChem | |
InChI Key |
BTBFCBQZFMQBNT-UHFFFAOYSA-N | |
Source | PubChem | |
URL | https://pubchem.ncbi.nlm.nih.gov | |
Description | Data deposited in or computed by PubChem | |
Canonical SMILES |
C1=CC(=C(C=C1C#N)F)F | |
Source | PubChem | |
URL | https://pubchem.ncbi.nlm.nih.gov | |
Description | Data deposited in or computed by PubChem | |
Molecular Formula |
C7H3F2N | |
Source | PubChem | |
URL | https://pubchem.ncbi.nlm.nih.gov | |
Description | Data deposited in or computed by PubChem | |
DSSTOX Substance ID |
DTXSID00214463 | |
Record name | 3,4-Difluorobenzonitrile | |
Source | EPA DSSTox | |
URL | https://comptox.epa.gov/dashboard/DTXSID00214463 | |
Description | DSSTox provides a high quality public chemistry resource for supporting improved predictive toxicology. | |
Molecular Weight |
139.10 g/mol | |
Source | PubChem | |
URL | https://pubchem.ncbi.nlm.nih.gov | |
Description | Data deposited in or computed by PubChem | |
CAS No. |
64248-62-0 | |
Record name | 3,4-Difluorobenzonitrile | |
Source | CAS Common Chemistry | |
URL | https://commonchemistry.cas.org/detail?cas_rn=64248-62-0 | |
Description | CAS Common Chemistry is an open community resource for accessing chemical information. Nearly 500,000 chemical substances from CAS REGISTRY cover areas of community interest, including common and frequently regulated chemicals, and those relevant to high school and undergraduate chemistry classes. This chemical information, curated by our expert scientists, is provided in alignment with our mission as a division of the American Chemical Society. | |
Explanation | The data from CAS Common Chemistry is provided under a CC-BY-NC 4.0 license, unless otherwise stated. | |
Record name | 3,4-Difluorobenzonitrile | |
Source | ChemIDplus | |
URL | https://pubchem.ncbi.nlm.nih.gov/substance/?source=chemidplus&sourceid=0064248620 | |
Description | ChemIDplus is a free, web search system that provides access to the structure and nomenclature authority files used for the identification of chemical substances cited in National Library of Medicine (NLM) databases, including the TOXNET system. | |
Record name | 3,4-Difluorobenzonitrile | |
Source | EPA DSSTox | |
URL | https://comptox.epa.gov/dashboard/DTXSID00214463 | |
Description | DSSTox provides a high quality public chemistry resource for supporting improved predictive toxicology. | |
Record name | 3,4-difluorobenzonitrile | |
Source | European Chemicals Agency (ECHA) | |
URL | https://echa.europa.eu/substance-information/-/substanceinfo/100.058.847 | |
Description | The European Chemicals Agency (ECHA) is an agency of the European Union which is the driving force among regulatory authorities in implementing the EU's groundbreaking chemicals legislation for the benefit of human health and the environment as well as for innovation and competitiveness. | |
Explanation | Use of the information, documents and data from the ECHA website is subject to the terms and conditions of this Legal Notice, and subject to other binding limitations provided for under applicable law, the information, documents and data made available on the ECHA website may be reproduced, distributed and/or used, totally or in part, for non-commercial purposes provided that ECHA is acknowledged as the source: "Source: European Chemicals Agency, http://echa.europa.eu/". Such acknowledgement must be included in each copy of the material. ECHA permits and encourages organisations and individuals to create links to the ECHA website under the following cumulative conditions: Links can only be made to webpages that provide a link to the Legal Notice page. | |
Retrosynthesis Analysis
AI-Powered Synthesis Planning: Our tool employs the Template_relevance Pistachio, Template_relevance Bkms_metabolic, Template_relevance Pistachio_ringbreaker, Template_relevance Reaxys, Template_relevance Reaxys_biocatalysis model, leveraging a vast database of chemical reactions to predict feasible synthetic routes.
One-Step Synthesis Focus: Specifically designed for one-step synthesis, it provides concise and direct routes for your target compounds, streamlining the synthesis process.
Accurate Predictions: Utilizing the extensive PISTACHIO, BKMS_METABOLIC, PISTACHIO_RINGBREAKER, REAXYS, REAXYS_BIOCATALYSIS database, our tool offers high-accuracy predictions, reflecting the latest in chemical research and data.
Strategy Settings
Precursor scoring | Relevance Heuristic |
---|---|
Min. plausibility | 0.01 |
Model | Template_relevance |
Template Set | Pistachio/Bkms_metabolic/Pistachio_ringbreaker/Reaxys/Reaxys_biocatalysis |
Top-N result to add to graph | 6 |
Feasible Synthetic Routes
Disclaimer and Information on In-Vitro Research Products
Please be aware that all articles and product information presented on BenchChem are intended solely for informational purposes. The products available for purchase on BenchChem are specifically designed for in-vitro studies, which are conducted outside of living organisms. In-vitro studies, derived from the Latin term "in glass," involve experiments performed in controlled laboratory settings using cells or tissues. It is important to note that these products are not categorized as medicines or drugs, and they have not received approval from the FDA for the prevention, treatment, or cure of any medical condition, ailment, or disease. We must emphasize that any form of bodily introduction of these products into humans or animals is strictly prohibited by law. It is essential to adhere to these guidelines to ensure compliance with legal and ethical standards in research and experimentation.