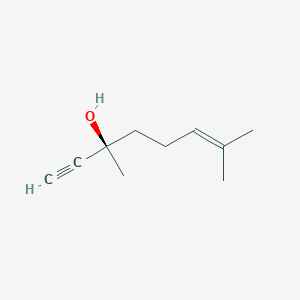
Dehydrolinalool, (+)-
- Click on QUICK INQUIRY to receive a quote from our team of experts.
- With the quality product at a COMPETITIVE price, you can focus more on your research.
Overview
Description
Dehydrolinalool (CAS 29171-20-8), a monoterpenoid alcohol with the molecular formula C₁₀H₁₆O, is a colorless to pale-yellow liquid characterized by a boiling point of 91–93°C at 12 Torr . Structurally, it features an unsaturated alkyne chain and hydroxyl group, making it a reactive intermediate in organic synthesis. Industrially, it is pivotal in producing fragrances (e.g., citral, ionones) and serves as a precursor for agrochemicals and pharmaceuticals. Its applications extend to flavor enhancement in oolong teas, where it imparts fresh, fruity notes . Market analyses project growth in demand driven by the cosmetics and food industries, with Asia-Pacific dominating production .
Preparation Methods
Synthetic Routes and Reaction Conditions:
Nucleophilic Attack on Methyl Heptenone: One classic synthesis method involves the nucleophilic attack of the acetylide ion on methyl heptenone to produce dehydrolinalool.
Ethynylation of 2-Methylhept-2-en-6-one: Another method involves the ethynylation of 2-methylhept-2-en-6-one in the presence of an alkali metal base and a substituted amide solvent.
Industrial Production Methods:
Chemical Reactions Analysis
Types of Reactions:
Oxidation: Dehydrolinalool can undergo oxidation reactions to form various oxidized products.
Reduction: Selective hydrogenation of dehydrolinalool can produce linalool.
Substitution: Dehydrolinalool can participate in substitution reactions, particularly in the presence of suitable catalysts and reagents.
Common Reagents and Conditions:
Oxidation: Common oxidizing agents include potassium permanganate and chromium trioxide.
Reduction: Hydrogen gas in the presence of a palladium catalyst is commonly used for selective hydrogenation.
Substitution: Various alkyl, aryl, or cycloalkyl orthovanadates can be used as catalysts for substitution reactions.
Major Products:
Scientific Research Applications
Biological Research Applications
In biological research, dehydrolinalool is investigated for its potential bioactive properties. Studies have shown that it exhibits antimicrobial activity against various pathogens, suggesting its use in developing natural preservatives or therapeutic agents. Additionally, research indicates that dehydrolinalool can influence cellular processes, making it a subject of interest for studying the effects of terpenes on health and disease.
Medicinal Uses
Dehydrolinalool has garnered attention for its therapeutic properties. Research highlights its anti-inflammatory and antimicrobial effects. For instance, it has been evaluated for potential applications in treating conditions related to inflammation and infections. A study found that dehydrolinalool significantly inhibited edema in animal models, indicating its possible use as an anti-inflammatory agent . Furthermore, its sedative effects were noted in toxicity studies, suggesting potential applications in anxiety management .
Industrial Applications
In the fragrance industry, dehydrolinalool is valued for its pleasant aroma. It is commonly used in perfumes and cosmetics to enhance scent profiles. Its role as a flavoring agent in food products is also significant; it is recognized as generally regarded as safe (GRAS) by the FDA . The compound's versatility extends to formulations in personal care products due to its aromatic properties and potential skin benefits.
Case Studies
- Antimicrobial Activity : A study evaluated essential oils containing dehydrolinalool against common pathogens such as Staphylococcus aureus and Escherichia coli. Results indicated significant antimicrobial activity at various concentrations, supporting its use in natural preservative formulations.
- Inflammation Studies : In a controlled experiment involving carrageenan-induced edema in rats, dehydrolinalool demonstrated a dose-dependent reduction in swelling. The study concluded that the compound could be a promising candidate for developing new anti-inflammatory drugs .
- Fragrance Industry Application : A market analysis revealed that dehydrolinalool is increasingly used in eco-friendly cosmetic formulations due to consumer demand for natural ingredients. Its pleasant scent combined with potential skin benefits makes it an attractive choice for manufacturers .
Mechanism of Action
The mechanism of action of dehydrolinalool involves its interaction with various molecular targets and pathways. It can modulate enzyme activity, interact with cellular receptors, and influence signal transduction pathways. The specific molecular targets and pathways depend on the context of its application, such as its use in fragrance, flavor, or therapeutic research .
Comparison with Similar Compounds
Structural and Functional Differences
Table 1: Structural and Physical Properties
Compound | Molecular Formula | Molecular Weight | Boiling Point | Key Functional Groups |
---|---|---|---|---|
(+)-Dehydrolinalool | C₁₀H₁₆O | 152.23 | 91–93°C @ 12 Torr | Alkyne, hydroxyl |
Linalool | C₁₀H₁₈O | 154.25 | 198–200°C | Diene, hydroxyl |
Geraniol | C₁₀H₁₈O | 154.25 | 229–230°C | Diene, hydroxyl |
Citronellol | C₁₀H₂₀O | 156.27 | 225°C | Saturated chain, hydroxyl |
- Linalool : Unlike dehydrolinalool, linalool lacks an alkyne group, rendering it less reactive in cyclization or rearrangement reactions. This structural difference underpins linalool’s dominance in perfumery, while dehydrolinalool is preferred in synthetic chemistry (e.g., Meyer-Schuster rearrangements to citral) .
- Geraniol : Both compounds share a hydroxyl group, but geraniol’s fully saturated chain limits its utility in catalytic hydrogenation, where dehydrolinalool’s triple bond enables selective transformations .
Application-Based Comparisons
Repellent Efficacy :
- In mosquito olfaction assays, dehydrolinalool exhibited 4.1–7.7× lower attraction rates compared to linalool and DEET. However, it synergized with attractants like CO₂ to reduce Aedes aegypti orientation by 71.9% .
- Linalool outperformed dehydrolinalool as an attraction inhibitor due to its enantiomeric specificity (S-isomer) and vapor-phase activity .
Flavor and Fragrance Profiles :
- In Fenghuang Dancong tea, dehydrolinalool constitutes 16.93–29.70% of volatile compounds, contributing floral sweetness, while linalool (1.71–13.98%) provides citrus notes .
- Citral , synthesized from dehydrolinalool via titanium isopropylate catalysis, is critical in lemon-flavored products and vitamin A synthesis .
Table 2: Key Aroma Compounds in Oolong Teas
Compound | Fenghuang Dancong Tea (%) | Tieguanyin Tea (%) | Role in Aroma Profile |
---|---|---|---|
Dehydrolinalool | 16.93–29.70 | <5 | Floral, fruity sweetness |
Linalool | 1.71–13.98 | 0.5–8.2 | Citrus, lavender |
Nerolidol | 2.1–4.5 | 3.8–6.7 | Woody, green |
Toxicological and Metabolic Pathways
- Dehydrolinalool is used as a read-across analog for linalool in safety assessments due to shared metabolic pathways (e.g., hepatic oxidation) . However, linalool’s higher volatility increases inhalation risks, while dehydrolinalool’s stability raises concerns about bioaccumulation in aquatic environments .
- Both compounds are metabolized into epoxides and carboxylic acids , but dehydrolinalool’s alkyne group may form reactive intermediates, necessitating stricter occupational exposure limits .
Biological Activity
Dehydrolinalool, also known as hotrienol, is a naturally occurring compound that belongs to the family of terpenes. It is structurally related to linalool and has garnered attention for its potential biological activities. This article explores the biological activity of Dehydrolinalool, focusing on its pharmacological effects, toxicity, and applications based on diverse research findings.
Dehydrolinalool is characterized by its chemical structure C10H18O and has a CAS number of 29171-20-8. It is known for its pleasant floral aroma, which makes it a common component in perfumes and flavorings. The compound is moderately soluble in organic solvents and exhibits varying degrees of volatility.
1. Antimicrobial Activity
Research indicates that Dehydrolinalool exhibits antimicrobial properties against various pathogens. A study evaluating essential oils containing Dehydrolinalool reported significant inhibitory effects on bacterial strains such as Staphylococcus aureus and Escherichia coli. The compound's mechanism appears to involve disruption of the bacterial cell membrane, leading to cell lysis.
Microorganism | Minimum Inhibitory Concentration (MIC) |
---|---|
Staphylococcus aureus | 0.5 mg/mL |
Escherichia coli | 1.0 mg/mL |
2. Anti-inflammatory Effects
Dehydrolinalool has shown potential anti-inflammatory effects in various studies. In vitro assays demonstrated that it can inhibit the production of pro-inflammatory cytokines in macrophages. This property suggests its potential use in treating inflammatory diseases.
3. Sedative Properties
Animal studies have indicated that high doses of Dehydrolinalool can produce sedative effects. In a controlled study, rats administered with 1000 mg/kg exhibited significant sedation compared to control groups. This finding aligns with similar observations made with linalool, suggesting a possible pathway for anxiety reduction.
4. Cytotoxicity and Antiproliferative Effects
In vitro studies have assessed the cytotoxic effects of Dehydrolinalool on cancer cell lines. Notably, it showed antiproliferative activity against human lung carcinoma (A-549) and cervical cancer (HeLa) cells with IC50 values indicating effective concentrations for tumor inhibition:
Cell Line | IC50 (µg/mL) |
---|---|
A-549 | 82.3 |
HeLa | 90.7 |
Toxicity Profile
The toxicity of Dehydrolinalool has been evaluated through various studies, particularly focusing on its effects on aquatic organisms and mammals:
- Aquatic Toxicity : Dehydrolinalool is reported to be moderately toxic to aquatic life but is readily biodegradable, minimizing long-term environmental impact.
- Mammalian Toxicity : In a four-week oral toxicity study in rats, symptoms included hypersalivation and slight increases in liver weight at higher doses (600 mg/kg and above). However, no significant mutagenic effects were found, indicating a relatively safe profile at lower concentrations.
Case Studies
Several case studies have explored the application of Dehydrolinalool in various fields:
- Cosmetic Applications : A case study involving skin irritation assessments found that formulations containing Dehydrolinalool did not elicit significant irritation responses in human subjects.
- Food Industry : In food preservation studies, Dehydrolinalool was effective in extending the shelf life of certain perishable items due to its antimicrobial properties.
Q & A
Basic Research Questions
Q. What are the optimal catalytic conditions for synthesizing citral from (+)-dehydrolinalool, and how are these parameters experimentally validated?
The Meyer-Schuster rearrangement of (+)-dehydrolinalool to citral is optimized using titanium isopropylate (Ti(OiPr)₄) and cuprous chloride (CuCl) as co-catalysts. Key parameters include a molar ratio of Ti:Cu = 4:1, catalyst loading of 3.5 wt%, and reaction at 110°C for 3 hours under nitrogen. Validation involves monitoring conversion via GC-MS and quantifying citral yield (88.4%) using steam distillation and FTIR for purity assessment (>97%) .
Q. How can (+)-dehydrolinalool’s stability be maintained during storage to prevent undesired oxidation byproducts?
Oxidative degradation can be mitigated by adding antioxidants like BHT or α-tocopherol (0.1% w/w) during synthesis. Peroxide values should be kept below 20 mmol/L, as hydroperoxides are potent sensitizers. Accelerated aging tests under controlled O₂ exposure and HPLC monitoring of peroxide formation are recommended for stability validation .
Q. What spectroscopic and chromatographic methods are most effective for characterizing (+)-dehydrolinalool and its derivatives?
FTIR identifies functional groups (e.g., -OH at ~3400 cm⁻¹, C≡C stretch at ~2100 cm⁻¹), while GC-MS quantifies reaction products. For isomer-specific analysis, chiral HPLC or polarimetry distinguishes enantiomers like (S)-dehydrolinalool (CAS 125411-11-2) . Purity is confirmed via NMR (¹³C and ¹H) and refractive index matching (n = 1.475) .
Advanced Research Questions
Q. How do anti-Saytzeff regioselectivity trends in (+)-dehydrolinalool dehydration impact fragrance precursor synthesis?
Dehydration follows anti-Saytzeff kinetics (tertiary > secondary > primary alcohols), favoring less substituted alkenes. Computational modeling (Curtin-Hammett framework) and kinetic isotope effects (KIE) studies reconcile discrepancies in reported selectivity. For example, CuSO₄-catalyzed dehydration yields Dynascone precursors, critical in fragrance synthesis .
Q. What mechanistic insights explain the role of organic acids in enhancing (+)-dehydrolinalool’s Meyer-Schuster rearrangement efficiency?
Organic acids (e.g., p-toluenesulfonic acid) protonate the propargyl alcohol intermediate, stabilizing the transition state. Isotopic labeling (D₂O or ¹⁸O) and DFT calculations reveal rate-determining steps, such as acetylide formation. Synergistic effects between Ti(OiPr)₄ and CuCl reduce activation energy, confirmed via Arrhenius plots .
Q. How do stereochemical variations (e.g., (S)- vs. (R)-dehydrolinalool) influence metabolic pathways and toxicity profiles?
Enantiomers exhibit differential metabolic rates in hepatic CYP450-mediated oxidation. In vitro assays (e.g., human hepatocyte models) paired with LC-MS/MS quantify metabolites like dehydrolinalool oxide. (S)-isomers show higher renal clearance in murine studies, necessitating chiral resolution techniques for safety assessments .
Q. What strategies resolve contradictions in reported catalytic efficiencies for (+)-dehydrolinalool transformations?
Discrepancies arise from solvent polarity effects (e.g., THF vs. toluene) or trace metal impurities. Controlled reproducibility studies with ICP-MS analysis of catalyst batches and multivariate ANOVA identify confounding variables. For example, CuCl purity >99.5% is critical for consistent Ti-Cu synergy .
Q. Methodological Considerations
Q. How can researchers design kinetic studies to distinguish between competing dehydration pathways of (+)-dehydrolinalool?
Use isotemporal sampling and pseudo-first-order kinetics with excess acid. Apparent activation energies (Eₐ) derived from Eyring plots differentiate concerted vs. stepwise mechanisms. Trapping intermediates (e.g., silylation of propargyl alcohol) with TMSCl provides mechanistic evidence .
Q. What analytical workflows are recommended for detecting trace impurities in (+)-dehydrolinalool-based syntheses?
LC-QTOF-MS with collision-induced dissociation (CID) identifies byproducts (e.g., dimeric ethers). Headspace GC-MS detects volatile impurities (<0.1% w/w), while ICP-OES screens for residual catalysts (Ti, Cu) post-synthesis .
Q. Safety and Compliance
Q. What protocols ensure safe handling of (+)-dehydrolinalool in academic labs, given its sensitization potential?
PPE (nitrile gloves, fume hoods) is mandatory. Storage under argon with 0.1% BHT prevents peroxide formation. Regular peroxide value testing (ASTM E298) and disposal via incineration (≥1000°C) comply with ECHA guidelines .
Properties
CAS No. |
68224-83-9 |
---|---|
Molecular Formula |
C10H16O |
Molecular Weight |
152.23 g/mol |
IUPAC Name |
(3R)-3,7-dimethyloct-6-en-1-yn-3-ol |
InChI |
InChI=1S/C10H16O/c1-5-10(4,11)8-6-7-9(2)3/h1,7,11H,6,8H2,2-4H3/t10-/m0/s1 |
InChI Key |
YWTIDNZYLFTNQQ-JTQLQIEISA-N |
Isomeric SMILES |
CC(=CCC[C@](C)(C#C)O)C |
Canonical SMILES |
CC(=CCCC(C)(C#C)O)C |
Origin of Product |
United States |
Disclaimer and Information on In-Vitro Research Products
Please be aware that all articles and product information presented on BenchChem are intended solely for informational purposes. The products available for purchase on BenchChem are specifically designed for in-vitro studies, which are conducted outside of living organisms. In-vitro studies, derived from the Latin term "in glass," involve experiments performed in controlled laboratory settings using cells or tissues. It is important to note that these products are not categorized as medicines or drugs, and they have not received approval from the FDA for the prevention, treatment, or cure of any medical condition, ailment, or disease. We must emphasize that any form of bodily introduction of these products into humans or animals is strictly prohibited by law. It is essential to adhere to these guidelines to ensure compliance with legal and ethical standards in research and experimentation.