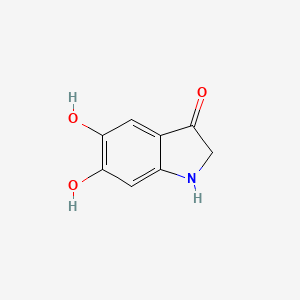
5,6-Dihydroxyindolin-3-one
- Click on QUICK INQUIRY to receive a quote from our team of experts.
- With the quality product at a COMPETITIVE price, you can focus more on your research.
Overview
Description
5,6-Dihydroxyindolin-3-one is an indole-derived heterocyclic compound characterized by a bicyclic structure consisting of a benzene ring fused to a pyrrolidone ring. Its molecular formula is $ \text{C}8\text{H}7\text{NO}_3 $, with hydroxyl (-OH) groups at positions 5 and 6 of the benzene ring and a ketone (=O) at position 3 of the pyrrolidone ring. This substitution pattern confers unique physicochemical properties, including high polarity due to the hydroxyl groups, which enhances solubility in polar solvents like water or ethanol.
Preparation Methods
Synthetic Routes and Reaction Conditions: A laboratory synthesis of 5,6-Dihydroxyindolin-3-one can be accomplished starting from 3,4-dibenzyloxybenzaldehyde . This compound is condensed with nitromethane in a Henry reaction, followed by nitration, reduction of the nitro groups, and hydrogenolysis of the benzyl protecting groups . Another method involves the use of 2,β-dinitrostyrene, disubstituted in positions 4 and 5, and corresponding to the formula: ##STR2## in which R denotes an acetoxy, benzyloxy, or hydroxy group . The compound is prepared by cyclizing reduction with hydrogen in the presence of a catalyst such as palladium on charcoal .
Industrial Production Methods: Industrial production methods for this compound involve the use of levodopa or its derivatives, solvent, and additive, followed by the addition of acid to dissolve the solute . The process also includes hydrogenation under pressure or hydrogen transfer operations in a solvent medium and in the presence of a hydrogenation catalyst .
Chemical Reactions Analysis
Types of Reactions: 5,6-Dihydroxyindolin-3-one undergoes various types of reactions, including oxidation, reduction, and substitution .
Common Reagents and Conditions: One-electron oxidation of this compound by the azide radical, N3˙, at pH 5 and 9 yields the benzosemiquinone radical . The presence of Zn2+ at pH 5.0 influences the reactions involving the semiquinone radical, forming a Zn ion complex of the o-semiquinone radical .
Major Products: The major products formed from these reactions include dopachrome-like intermediates and Zn2+–quinone complexes .
Scientific Research Applications
Synthesis and Production
The production of 5,6-dihydroxyindoline can be achieved through several synthetic routes. A notable method involves the ether cleavage of 5,6-dimethoxyindoline using hydrobromic acid, followed by direct crystallization from the reaction mixture. This method has been highlighted for its efficiency in yielding the compound suitable for further applications .
Biological Activities
Antiviral and Antiparasitic Properties
Research has indicated that 5,6-dihydroxyindole and its derivatives exhibit cytotoxic effects against various pathogens. Studies demonstrate that these compounds can kill selected bacteria and fungi, showcasing their potential as antimicrobial agents . Additionally, they have been shown to possess antiviral properties against baculoviruses, suggesting their application in virology and pest control .
Melanin Precursor
5,6-Dihydroxyindoline serves as a precursor in the biosynthesis of melanin. This property is particularly relevant in the context of developing natural hair dyes. The oxidative polymerization of 5,6-dihydroxyindole leads to the formation of melanins, which are utilized in cosmetic formulations for hair coloring . However, the instability of 5,6-dihydroxyindole in aqueous solutions poses challenges for its use in hair dye preparations.
Therapeutic Applications
Cancer Research
Emerging studies suggest that compounds related to 5,6-dihydroxyindoline may have applications in cancer therapy. For instance, modifications of this compound have shown promising inhibitory activity against specific cancer cell lines. The ability to selectively target cancer cells while sparing normal cells highlights its potential as a therapeutic agent .
Anti-Tubercular Activity
Recent research has explored the anti-tubercular properties of nitrogen-containing derivatives related to 5,6-dihydroxyindoline. These compounds exhibited significant inhibitory activity against Mycobacterium tuberculosis, indicating their potential role in developing new anti-tubercular drugs .
Case Studies
- Hair Dye Formulations : A study evaluated the incorporation of 5,6-dihydroxyindole in hair dye products at varying concentrations. Results indicated effective coloring with minimal adverse reactions when used at recommended levels .
- Antiviral Studies : In experiments assessing the virucidal activity of 5,6-dihydroxyindole against baculoviruses, treated viral stocks showed a significant reduction in infectivity compared to controls. This underscores its potential utility in antiviral applications .
Mechanism of Action
The mechanism of action of 5,6-Dihydroxyindolin-3-one involves its conversion into indole-5,6-quinone through a reaction catalyzed by a tyrosinase enzyme . This compound acts as an intermediate in the production of eumelanin, which is involved in various biological processes . The molecular targets and pathways involved include the formation of benzosemiquinone radicals and their subsequent reactions to form dopachrome-like intermediates and Zn2+–quinone complexes .
Comparison with Similar Compounds
Comparison with Structurally Similar Compounds
The following analysis compares 5,6-Dihydroxyindolin-3-one with substituted indolinones and related derivatives, focusing on structural variations, substituent effects, and inferred properties.
5,6-Difluoroindolin-2-one
- Structure : Fluorine atoms replace hydroxyl groups at positions 5 and 6, with a ketone at position 2.
- Key Differences :
- Polarity : Fluorine’s electronegativity increases polarity but lacks hydrogen-bonding capacity, reducing solubility in polar solvents compared to this compound.
- Lipophilicity : Greater lipophilicity due to fluorine substitution may enhance membrane permeability, making it more suitable for biological applications requiring cellular uptake .
- Synthesis : Likely synthesized via electrophilic fluorination of indolin-2-one precursors.
5,6-Difluoroisatin (5,6-Difluoroindolin-2,3-dione)
- Structure : Contains two fluorine atoms at positions 5 and 6, with dual ketone groups at positions 2 and 3.
- Key Differences :
6-Hydroxy-3-(hydroxyimino)indolin-2-one
- Structure: Features a hydroxyl group at position 6 and a hydroxyimino (-N-OH) group at position 3.
- Key Differences: Chelation Potential: The hydroxyimino group enables metal ion chelation, a property absent in this compound. Stability: Crystallographic studies indicate planar geometry and strong intermolecular hydrogen bonding, suggesting higher thermal stability .
4,5,6,7-Tetrachloro-1,2-dihydro-3H-indol-3-one
- Structure : Chlorine atoms at positions 4–7 and a saturated pyrrolidine ring.
- Key Differences: Electron-Withdrawing Effects: Chlorine’s strong electron-withdrawing nature deactivates the aromatic ring, reducing electrophilic substitution reactivity compared to hydroxylated analogs.
5,7-Difluoroindolin-2-one
- Structure : Fluorine atoms at positions 5 and 7, with a ketone at position 2.
- Biological Activity: Fluorine’s metabolic resistance may enhance in vivo stability compared to hydroxylated derivatives .
Research Implications and Limitations
- Gaps in Data : The evidence lacks direct studies on this compound’s synthesis, stability, or bioactivity. Comparisons rely on chemical principles and analogs like fluorinated or chlorinated derivatives .
- Functional Group Impact : Hydroxyl groups favor solubility and H-bonding but may limit metabolic stability. Halogenation enhances lipophilicity and durability but introduces environmental concerns.
- Future Directions : Experimental validation of redox behavior, chelation efficacy, and pharmacokinetics is needed to advance applications in pharmacology or materials science.
Biological Activity
5,6-Dihydroxyindolin-3-one (DHI) is a significant compound in the realm of biological research, particularly due to its diverse biological activities. This article delves into its antibacterial, antifungal, antiviral, and antiparasitic properties, as well as its cytotoxic effects on various cell types. The findings are supported by case studies and data tables that summarize key research outcomes.
This compound is a derivative of indole and is primarily known for its role as an intermediate in the biosynthesis of eumelanin. It can be synthesized through various methods, including the oxidation of dopamine to norepichrome followed by reduction to leuco compounds and subsequent transformations to yield DHI . The molecular formula for DHI is C8H7NO2.
Antibacterial and Antifungal Activity
DHI exhibits broad-spectrum antibacterial and antifungal activities. Research indicates that it can effectively inhibit the growth of various bacterial strains and fungi. For instance, a study demonstrated that DHI's spontaneous oxidation products possess significant antibacterial properties against pathogens such as Escherichia coli and Staphylococcus aureus, with minimum inhibitory concentrations (MICs) reported in the micromolar range .
Table 1: Antibacterial Activity of this compound
Pathogen | MIC (µM) |
---|---|
E. coli | 15 |
S. aureus | 20 |
Candida albicans | 25 |
Antiviral and Antiparasitic Effects
DHI has shown promising antiviral properties, particularly against baculoviruses. In vitro studies revealed that preincubation with DHI significantly reduced viral infectivity in insect cell lines. For example, an experiment reported a 97% mortality rate in Sf9 cells treated with DHI at a concentration of 1 mM . Additionally, DHI demonstrated activity against parasitic wasps, with LC50 values indicating effective toxicity towards their eggs .
Table 2: Virucidal Activity of this compound
Virus/Parasite | LC50 (µM) |
---|---|
Baculovirus | 5.6 |
Microplitis demolitor eggs | 111.0 |
Cytotoxic Effects
While DHI exhibits beneficial antimicrobial properties, it also poses cytotoxic risks to host cells. Studies have shown that high concentrations of DHI can lead to DNA polymerization and protein crosslinking in human cells, suggesting potential implications for cellular health . Notably, the cytotoxicity is dose-dependent; lower concentrations may be safe while higher doses can lead to significant cellular damage.
Table 3: Cytotoxicity of this compound on Human Cells
Concentration (mM) | Cell Viability (%) |
---|---|
0.1 | 90 |
0.5 | 75 |
1.0 | 50 |
2.0 | <10 |
Case Studies
- Insect Immunity : A study investigated the role of DHI in insect immune responses, particularly through the activation of phenoloxidase (PO). The research highlighted how DHI production upon infection aids in pathogen immobilization but also risks damaging host tissues if not regulated properly .
- Human Retinal Cells : Another study assessed the effects of DHI on human retinal pigment epithelial cells (ARPE-19). Results indicated that exposure to DHI could exacerbate UV-A induced damage, raising concerns about its safety in ocular applications .
Q & A
Q. Basic: What are the established synthesis protocols for 5,6-Dihydroxyindolin-3-one, and how can purity be optimized?
Methodological Answer:
Synthesis typically involves oxidative cyclization of precursor indole derivatives under controlled pH and temperature. Solvent systems like ethanol/water mixtures are preferred to stabilize hydroxyl groups . Purification methods include reverse-phase HPLC or column chromatography using silica gel with ethyl acetate/hexane gradients. Purity validation requires NMR (1H/13C) and high-resolution mass spectrometry (HRMS) to confirm structural integrity and rule out byproducts .
Q. Advanced: How can mechanistic pathways for this compound’s reactivity be elucidated experimentally?
Methodological Answer:
Kinetic isotope effect (KIE) studies and trapping of reactive intermediates (e.g., using TEMPO for radical detection) are critical. Time-resolved spectroscopy (UV-Vis, fluorescence) under varying oxygen concentrations can reveal oxidation kinetics. Computational modeling (DFT) paired with experimental data validates proposed mechanisms .
Q. Basic: What analytical techniques are most reliable for characterizing this compound’s stability under physiological conditions?
Methodological Answer:
Stability assays in buffers (pH 4–9) at 37°C, monitored via LC-MS over 24–72 hours, identify degradation products. Accelerated stability studies (e.g., 40°C/75% RH) combined with FTIR track hydroxyl group oxidation. Storage recommendations: anhydrous conditions at -20°C in amber vials to prevent photodegradation .
Q. Advanced: How should researchers address contradictions in reported biological activities of this compound derivatives?
Methodological Answer:
Systematic meta-analysis of existing literature (e.g., PRISMA guidelines) to identify variables like assay conditions, cell lines, or solvent effects. Replicate key studies under standardized protocols (e.g., OECD guidelines for cytotoxicity) to isolate confounding factors. Use dose-response curves and statistical tools (ANOVA, Bland-Altman plots) to quantify discrepancies .
Q. Basic: What in vitro models are suitable for preliminary toxicity screening of this compound?
Methodological Answer:
Use immortalized cell lines (e.g., HEK293, HepG2) for cytotoxicity (MTT assay) and genotoxicity (Comet assay). Include positive controls (e.g., hydrogen peroxide for oxidative stress). For receptor-binding studies, SPR (surface plasmon resonance) quantifies affinity for targets like melanocortin receptors .
Q. Advanced: How can computational tools predict this compound’s electronic properties for material science applications?
Methodological Answer:
Density Functional Theory (DFT) calculations (B3LYP/6-311++G** basis set) model HOMO-LUMO gaps and redox potentials. Molecular dynamics simulations (AMBER/CHARMM) assess solubility and aggregation behavior. Validate predictions against experimental X-ray crystallography data (e.g., bond lengths, dihedral angles) .
Q. Basic: What guidelines ensure rigorous data presentation in publications on this compound?
Methodological Answer:
Follow journal-specific standards (e.g., Medicinal Chemistry Research):
- Tables: Include yield, purity, and spectroscopic data (δ ppm for NMR, m/z for HRMS).
- Figures: Use line graphs for kinetic data and heatmaps for dose-dependent effects. Avoid duplicating data in text and visuals .
- Supplemental Information: Raw crystallographic data (CIF files) and HPLC chromatograms .
Q. Advanced: How to design a research proposal for studying this compound’s role in neurodegenerative pathways?
Methodological Answer:
- Hypothesis: “this compound modulates α-synuclein aggregation via redox interactions.”
- Methods:
- In vitro: Thioflavin T assays for fibril formation; TEM for morphology.
- In vivo: Transgenic C. elegans models (e.g., NL5901 strain) treated with 0.1–10 µM compound.
- Controls: Include antioxidants (e.g., ascorbate) and knockout strains .
Q. Basic: What are the best practices for literature reviews on this compound’s pharmacological potential?
Methodological Answer:
Use databases (PubMed, SciFinder) with keywords: “indolinone derivatives,” “redox-active heterocycles,” “neuroprotective agents.” Critically appraise sources using PRISMA flowcharts, prioritizing peer-reviewed studies over patents. Track citation networks (e.g., Web of Science) to identify seminal works .
Q. Advanced: How to integrate this compound into cross-disciplinary research (e.g., materials chemistry vs. medicinal chemistry)?
Methodological Answer:
- Materials Science: Study its semiconductor properties via cyclic voltammetry and bandgap measurements.
- Medicinal Chemistry: Optimize bioavailability via prodrug design (e.g., acetyl-protected hydroxyl groups).
- Collaborative Framework: Use shared datasets (e.g., Cambridge Structural Database) and joint authorship to align objectives .
Properties
Molecular Formula |
C8H7NO3 |
---|---|
Molecular Weight |
165.15 g/mol |
IUPAC Name |
5,6-dihydroxy-1,2-dihydroindol-3-one |
InChI |
InChI=1S/C8H7NO3/c10-6-1-4-5(2-7(6)11)9-3-8(4)12/h1-2,9-11H,3H2 |
InChI Key |
ZVDJZAGLPBLUHM-UHFFFAOYSA-N |
Canonical SMILES |
C1C(=O)C2=CC(=C(C=C2N1)O)O |
Origin of Product |
United States |
Disclaimer and Information on In-Vitro Research Products
Please be aware that all articles and product information presented on BenchChem are intended solely for informational purposes. The products available for purchase on BenchChem are specifically designed for in-vitro studies, which are conducted outside of living organisms. In-vitro studies, derived from the Latin term "in glass," involve experiments performed in controlled laboratory settings using cells or tissues. It is important to note that these products are not categorized as medicines or drugs, and they have not received approval from the FDA for the prevention, treatment, or cure of any medical condition, ailment, or disease. We must emphasize that any form of bodily introduction of these products into humans or animals is strictly prohibited by law. It is essential to adhere to these guidelines to ensure compliance with legal and ethical standards in research and experimentation.