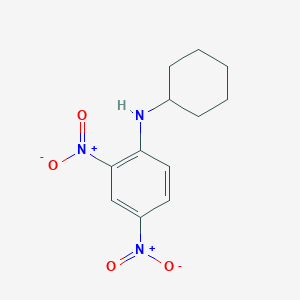
N-cyclohexyl-2,4-dinitroaniline
Overview
Description
N-cyclohexyl-2,4-dinitroaniline is an organic compound with the molecular formula C₁₂H₁₅N₃O₄. It is a derivative of aniline and dinitrobenzene, characterized by the presence of a cyclohexyl group attached to the nitrogen atom of the aniline ring. This compound is known for its applications in various fields, including agriculture, pharmaceuticals, and materials science .
Mechanism of Action
Target of Action
N-cyclohexyl-2,4-dinitroaniline, like other dinitroanilines, primarily targets tubulin proteins in plants and protists . Tubulin proteins are crucial for the formation of microtubules, which are essential components of the cell’s cytoskeleton. They play a vital role in cell division, intracellular transport, and maintaining cell shape .
Mode of Action
This compound acts as a microtubule inhibitor . It binds to tubulin proteins, preventing their polymerization into microtubules. This disruption of microtubule formation inhibits cell division, particularly mitosis, leading to cell death . Target-site resistance (TSR) to dinitroaniline herbicides due to point mutations in α-tubulin genes has been confirmed in a few weedy plant species .
Biochemical Pathways
The primary biochemical pathway affected by this compound is the microtubule assembly pathway . By inhibiting the polymerization of tubulin into microtubules, the compound disrupts the normal functioning of this pathway. This disruption can lead to various downstream effects, including impaired cell division and growth, ultimately leading to cell death .
Pharmacokinetics
Based on the properties of similar dinitroaniline compounds, it can be inferred that these compounds are likely to be absorbed by the roots due to their proximity to the herbicide, but translocation from the root to the top is minimal .
Result of Action
The primary result of this compound’s action is the inhibition of cell division , particularly mitosis, leading to cell death . This is due to the compound’s inhibitory effect on microtubule formation, which is essential for cell division. In plants, this can lead to stunted growth and eventually plant death .
Preparation Methods
Synthetic Routes and Reaction Conditions: N-cyclohexyl-2,4-dinitroaniline can be synthesized through the nitration of N-cyclohexylaniline. The process involves the reaction of N-cyclohexylaniline with a nitrating agent, typically a mixture of concentrated sulfuric acid and nitric acid, under controlled temperature conditions to yield the dinitro compound .
Industrial Production Methods: In industrial settings, the production of this compound often involves the use of continuous flow reactors to ensure consistent quality and yield. The reaction conditions are optimized to minimize by-products and maximize the efficiency of the nitration process .
Chemical Reactions Analysis
Types of Reactions: N-cyclohexyl-2,4-dinitroaniline undergoes various chemical reactions, including:
Reduction: The nitro groups can be reduced to amino groups using reducing agents such as hydrogen gas in the presence of a catalyst like palladium on carbon.
Common Reagents and Conditions:
Reduction: Hydrogen gas, palladium on carbon catalyst.
Substitution: Nucleophiles such as amines or thiols, often in the presence of a base.
Major Products Formed:
Reduction: N-cyclohexyl-2,4-diaminoaniline.
Substitution: Various substituted aniline derivatives depending on the nucleophile used.
Scientific Research Applications
N-cyclohexyl-2,4-dinitroaniline has several applications in scientific research:
Chemistry: Used as an intermediate in the synthesis of dyes and pigments.
Biology: Studied for its potential as a bioactive compound with antimicrobial properties.
Medicine: Investigated for its potential use in the development of pharmaceuticals.
Industry: Utilized in the production of herbicides and other agrochemicals
Comparison with Similar Compounds
- 2,4-Dinitroaniline
- 2,5-Dinitroaniline
- 2,6-Dinitroaniline
- 3,4-Dinitroaniline
- 3,5-Dinitroaniline
Comparison: N-cyclohexyl-2,4-dinitroaniline is unique due to the presence of the cyclohexyl group, which imparts distinct chemical and physical properties compared to other dinitroaniline derivatives. This structural difference can influence its reactivity, solubility, and biological activity .
Properties
IUPAC Name |
N-cyclohexyl-2,4-dinitroaniline | |
---|---|---|
Source | PubChem | |
URL | https://pubchem.ncbi.nlm.nih.gov | |
Description | Data deposited in or computed by PubChem | |
InChI |
InChI=1S/C12H15N3O4/c16-14(17)10-6-7-11(12(8-10)15(18)19)13-9-4-2-1-3-5-9/h6-9,13H,1-5H2 | |
Source | PubChem | |
URL | https://pubchem.ncbi.nlm.nih.gov | |
Description | Data deposited in or computed by PubChem | |
InChI Key |
CGNCMUWOBHOSEQ-UHFFFAOYSA-N | |
Source | PubChem | |
URL | https://pubchem.ncbi.nlm.nih.gov | |
Description | Data deposited in or computed by PubChem | |
Canonical SMILES |
C1CCC(CC1)NC2=C(C=C(C=C2)[N+](=O)[O-])[N+](=O)[O-] | |
Source | PubChem | |
URL | https://pubchem.ncbi.nlm.nih.gov | |
Description | Data deposited in or computed by PubChem | |
Molecular Formula |
C12H15N3O4 | |
Source | PubChem | |
URL | https://pubchem.ncbi.nlm.nih.gov | |
Description | Data deposited in or computed by PubChem | |
DSSTOX Substance ID |
DTXSID20323445 | |
Record name | N-cyclohexyl-2,4-dinitroaniline | |
Source | EPA DSSTox | |
URL | https://comptox.epa.gov/dashboard/DTXSID20323445 | |
Description | DSSTox provides a high quality public chemistry resource for supporting improved predictive toxicology. | |
Molecular Weight |
265.26 g/mol | |
Source | PubChem | |
URL | https://pubchem.ncbi.nlm.nih.gov | |
Description | Data deposited in or computed by PubChem | |
CAS No. |
52790-66-6 | |
Record name | NSC404031 | |
Source | DTP/NCI | |
URL | https://dtp.cancer.gov/dtpstandard/servlet/dwindex?searchtype=NSC&outputformat=html&searchlist=404031 | |
Description | The NCI Development Therapeutics Program (DTP) provides services and resources to the academic and private-sector research communities worldwide to facilitate the discovery and development of new cancer therapeutic agents. | |
Explanation | Unless otherwise indicated, all text within NCI products is free of copyright and may be reused without our permission. Credit the National Cancer Institute as the source. | |
Record name | N-cyclohexyl-2,4-dinitroaniline | |
Source | EPA DSSTox | |
URL | https://comptox.epa.gov/dashboard/DTXSID20323445 | |
Description | DSSTox provides a high quality public chemistry resource for supporting improved predictive toxicology. | |
Synthesis routes and methods
Procedure details
Retrosynthesis Analysis
AI-Powered Synthesis Planning: Our tool employs the Template_relevance Pistachio, Template_relevance Bkms_metabolic, Template_relevance Pistachio_ringbreaker, Template_relevance Reaxys, Template_relevance Reaxys_biocatalysis model, leveraging a vast database of chemical reactions to predict feasible synthetic routes.
One-Step Synthesis Focus: Specifically designed for one-step synthesis, it provides concise and direct routes for your target compounds, streamlining the synthesis process.
Accurate Predictions: Utilizing the extensive PISTACHIO, BKMS_METABOLIC, PISTACHIO_RINGBREAKER, REAXYS, REAXYS_BIOCATALYSIS database, our tool offers high-accuracy predictions, reflecting the latest in chemical research and data.
Strategy Settings
Precursor scoring | Relevance Heuristic |
---|---|
Min. plausibility | 0.01 |
Model | Template_relevance |
Template Set | Pistachio/Bkms_metabolic/Pistachio_ringbreaker/Reaxys/Reaxys_biocatalysis |
Top-N result to add to graph | 6 |
Feasible Synthetic Routes
Disclaimer and Information on In-Vitro Research Products
Please be aware that all articles and product information presented on BenchChem are intended solely for informational purposes. The products available for purchase on BenchChem are specifically designed for in-vitro studies, which are conducted outside of living organisms. In-vitro studies, derived from the Latin term "in glass," involve experiments performed in controlled laboratory settings using cells or tissues. It is important to note that these products are not categorized as medicines or drugs, and they have not received approval from the FDA for the prevention, treatment, or cure of any medical condition, ailment, or disease. We must emphasize that any form of bodily introduction of these products into humans or animals is strictly prohibited by law. It is essential to adhere to these guidelines to ensure compliance with legal and ethical standards in research and experimentation.