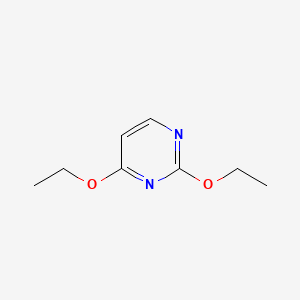
2,4-Diethoxypyrimidine
Overview
Description
2,4-Diethoxypyrimidine is an organic compound with the chemical formula C10H14N2O2. It is a derivative of pyrimidine, a heterocyclic aromatic organic compound similar to pyridine. Pyrimidine derivatives are significant in various fields, including pharmaceuticals, agrochemicals, and materials science, due to their diverse biological activities and chemical properties.
Scientific Research Applications
2,4-Diethoxypyrimidine has several applications in scientific research, including:
Chemistry: Used as a building block for the synthesis of more complex pyrimidine derivatives.
Biology: Studied for its potential biological activities, including antimicrobial and anticancer properties.
Medicine: Investigated for its potential use in drug development, particularly as a scaffold for designing new therapeutic agents.
Industry: Utilized in the production of agrochemicals and materials with specific properties.
Safety and Hazards
The safety data sheet for 2,4-Diethoxypyrimidine advises avoiding dust formation, breathing mist, gas or vapours, and contacting with skin and eye . It recommends using personal protective equipment, wearing chemical impermeable gloves, ensuring adequate ventilation, and removing all sources of ignition .
Mechanism of Action
Target of Action
The primary target of 2,4-Diethoxypyrimidine is myofibrillar proteins (MP) in grouper (Epinephelus coioides), a type of fish . These proteins play a crucial role in muscle contraction and are essential for the fish’s movement and overall health.
Mode of Action
This compound, being a lipid oxidation product, interacts with these myofibrillar proteins, altering their conformation and inducing oxidative degradation . This interaction is primarily driven by hydrophobic interactions and hydrogen bonding .
Biochemical Pathways
The interaction of this compound with myofibrillar proteins affects several metabolic pathways. Key volatile compounds (VOCs) associated with the overall flavor of MP were identified, suggesting that the metabolism of arginine, proline, alanine, aspartate, glutamate, and beta-alanine is affected . These compounds could potentially be products of arginine biosynthesis .
Result of Action
The result of this compound’s action is a change in the conformation of myofibrillar proteins, leading to oxidative degradation . This can affect the overall aroma development of the grouper, influencing its flavor during storage .
Action Environment
The action of this compound is influenced by environmental factors such as temperature and the presence of oxygen, which can affect the rate of lipid oxidation and, consequently, the production of this compound . The stability and efficacy of this compound may also be affected by these factors.
Preparation Methods
Synthetic Routes and Reaction Conditions
The synthesis of 2,4-Diethoxypyrimidine typically involves the reaction of ethyl orthoformate with urea under acidic conditions. The process can be summarized as follows:
Condensation Reaction: Ethyl orthoformate reacts with urea in the presence of an acid catalyst, such as hydrochloric acid, to form this compound.
Reaction Conditions: The reaction is usually carried out at elevated temperatures (around 100-150°C) to facilitate the condensation process.
Industrial Production Methods
In an industrial setting, the production of this compound may involve continuous flow reactors to ensure consistent quality and yield. The use of automated systems allows for precise control of reaction parameters, such as temperature, pressure, and reactant concentrations, leading to efficient large-scale production.
Chemical Reactions Analysis
Types of Reactions
2,4-Diethoxypyrimidine undergoes various chemical reactions, including:
Nucleophilic Substitution: The ethoxy groups can be replaced by other nucleophiles, such as amines or thiols, under appropriate conditions.
Oxidation: The compound can be oxidized to form corresponding pyrimidine derivatives with different functional groups.
Reduction: Reduction reactions can convert this compound into its corresponding dihydropyrimidine derivatives.
Common Reagents and Conditions
Nucleophilic Substitution: Common reagents include amines, thiols, and alkoxides. The reactions are typically carried out in polar solvents like ethanol or dimethylformamide at moderate temperatures (50-100°C).
Oxidation: Oxidizing agents such as potassium permanganate or hydrogen peroxide are used under acidic or basic conditions.
Reduction: Reducing agents like sodium borohydride or lithium aluminum hydride are employed under anhydrous conditions.
Major Products Formed
Nucleophilic Substitution: Substituted pyrimidine derivatives with various functional groups.
Oxidation: Oxidized pyrimidine derivatives with hydroxyl or carbonyl groups.
Reduction: Dihydropyrimidine derivatives with reduced double bonds.
Comparison with Similar Compounds
Similar Compounds
2,4-Dimethoxypyrimidine: Similar structure but with methoxy groups instead of ethoxy groups.
2,4-Diaminopyrimidine: Contains amino groups instead of ethoxy groups.
2,4-Dichloropyrimidine: Contains chlorine atoms instead of ethoxy groups.
Uniqueness
2,4-Diethoxypyrimidine is unique due to its ethoxy substituents, which confer specific chemical and physical properties, such as solubility and reactivity, making it suitable for particular applications in synthesis and research.
Properties
IUPAC Name |
2,4-diethoxypyrimidine | |
---|---|---|
Source | PubChem | |
URL | https://pubchem.ncbi.nlm.nih.gov | |
Description | Data deposited in or computed by PubChem | |
InChI |
InChI=1S/C8H12N2O2/c1-3-11-7-5-6-9-8(10-7)12-4-2/h5-6H,3-4H2,1-2H3 | |
Source | PubChem | |
URL | https://pubchem.ncbi.nlm.nih.gov | |
Description | Data deposited in or computed by PubChem | |
InChI Key |
REZCUNDZKVUACL-UHFFFAOYSA-N | |
Source | PubChem | |
URL | https://pubchem.ncbi.nlm.nih.gov | |
Description | Data deposited in or computed by PubChem | |
Canonical SMILES |
CCOC1=NC(=NC=C1)OCC | |
Source | PubChem | |
URL | https://pubchem.ncbi.nlm.nih.gov | |
Description | Data deposited in or computed by PubChem | |
Molecular Formula |
C8H12N2O2 | |
Source | PubChem | |
URL | https://pubchem.ncbi.nlm.nih.gov | |
Description | Data deposited in or computed by PubChem | |
DSSTOX Substance ID |
DTXSID80942636 | |
Record name | 2,4-Diethoxypyrimidinato | |
Source | EPA DSSTox | |
URL | https://comptox.epa.gov/dashboard/DTXSID80942636 | |
Description | DSSTox provides a high quality public chemistry resource for supporting improved predictive toxicology. | |
Molecular Weight |
168.19 g/mol | |
Source | PubChem | |
URL | https://pubchem.ncbi.nlm.nih.gov | |
Description | Data deposited in or computed by PubChem | |
CAS No. |
20461-60-3 | |
Record name | NSC 13689 | |
Source | ChemIDplus | |
URL | https://pubchem.ncbi.nlm.nih.gov/substance/?source=chemidplus&sourceid=0020461603 | |
Description | ChemIDplus is a free, web search system that provides access to the structure and nomenclature authority files used for the identification of chemical substances cited in National Library of Medicine (NLM) databases, including the TOXNET system. | |
Record name | 20461-60-3 | |
Source | DTP/NCI | |
URL | https://dtp.cancer.gov/dtpstandard/servlet/dwindex?searchtype=NSC&outputformat=html&searchlist=13689 | |
Description | The NCI Development Therapeutics Program (DTP) provides services and resources to the academic and private-sector research communities worldwide to facilitate the discovery and development of new cancer therapeutic agents. | |
Explanation | Unless otherwise indicated, all text within NCI products is free of copyright and may be reused without our permission. Credit the National Cancer Institute as the source. | |
Record name | 2,4-Diethoxypyrimidinato | |
Source | EPA DSSTox | |
URL | https://comptox.epa.gov/dashboard/DTXSID80942636 | |
Description | DSSTox provides a high quality public chemistry resource for supporting improved predictive toxicology. | |
Retrosynthesis Analysis
AI-Powered Synthesis Planning: Our tool employs the Template_relevance Pistachio, Template_relevance Bkms_metabolic, Template_relevance Pistachio_ringbreaker, Template_relevance Reaxys, Template_relevance Reaxys_biocatalysis model, leveraging a vast database of chemical reactions to predict feasible synthetic routes.
One-Step Synthesis Focus: Specifically designed for one-step synthesis, it provides concise and direct routes for your target compounds, streamlining the synthesis process.
Accurate Predictions: Utilizing the extensive PISTACHIO, BKMS_METABOLIC, PISTACHIO_RINGBREAKER, REAXYS, REAXYS_BIOCATALYSIS database, our tool offers high-accuracy predictions, reflecting the latest in chemical research and data.
Strategy Settings
Precursor scoring | Relevance Heuristic |
---|---|
Min. plausibility | 0.01 |
Model | Template_relevance |
Template Set | Pistachio/Bkms_metabolic/Pistachio_ringbreaker/Reaxys/Reaxys_biocatalysis |
Top-N result to add to graph | 6 |
Feasible Synthetic Routes
Q1: What is the main synthetic utility of 2,4-Diethoxypyrimidine?
A1: this compound serves as a versatile intermediate in synthesizing various substituted pyrimidines. This utility arises from the reactivity of the ethoxy groups, which can be selectively replaced by other functional groups. For instance, reactions with nucleophiles can lead to the displacement of one or both ethoxy groups, providing access to diverse pyrimidine derivatives [, , , ].
Q2: Can you provide an example of a specific reaction pathway using this compound as a starting material?
A2: Absolutely. In a study focusing on synthesizing potential antiviral agents [], researchers utilized this compound to prepare 1-[(2-hydroxyethoxy)methyl]uracil (HEMU). This involved reacting this compound with 2-(benzoyloxy)ethoxymethyl chloride, followed by a hydrolysis step to yield the desired HEMU. This exemplifies how the reactivity of this compound allows for the introduction of specific substituents onto the pyrimidine ring.
Q3: The abstracts mention the "Hilbert-Johnson reaction." What is the significance of this reaction in the context of this compound?
A3: The Hilbert–Johnson reaction [, ] is a key synthetic tool when using this compound. This reaction typically involves the condensation of this compound with α-halo carbonyl compounds, like α-bromo-γ-butyrolactone []. This reaction allows for the formation of a new carbon-carbon bond, effectively adding a substituent to the pyrimidine ring. Subsequent modifications of this newly attached group can then lead to a variety of pyrimidine analogs.
Q4: Are there any limitations to using this compound in synthesis?
A4: While a valuable starting material, this compound does present some challenges. Researchers noted that the Hilbert-Johnson reaction, while useful, isn't always successful. For instance, less reactive substrates like 5-bromovaleronitrile showed poor reactivity with this compound []. This highlights the need for carefully considering the reactivity of both the pyrimidine derivative and the intended reaction partner.
Q5: The research focuses on creating new molecules. Are there any insights into the analytical techniques used to characterize the synthesized compounds?
A5: While the abstracts don't delve deeply into specific analytical methodologies, they do mention using UV spectroscopy to characterize the synthesized compounds []. This technique is particularly useful in analyzing pyrimidine derivatives, as they absorb strongly in the UV region. Changes in the UV spectra can provide information about the structure and purity of the synthesized molecules. Further characterization likely involved techniques like NMR and mass spectrometry, standard tools in organic synthesis for structural elucidation.
Disclaimer and Information on In-Vitro Research Products
Please be aware that all articles and product information presented on BenchChem are intended solely for informational purposes. The products available for purchase on BenchChem are specifically designed for in-vitro studies, which are conducted outside of living organisms. In-vitro studies, derived from the Latin term "in glass," involve experiments performed in controlled laboratory settings using cells or tissues. It is important to note that these products are not categorized as medicines or drugs, and they have not received approval from the FDA for the prevention, treatment, or cure of any medical condition, ailment, or disease. We must emphasize that any form of bodily introduction of these products into humans or animals is strictly prohibited by law. It is essential to adhere to these guidelines to ensure compliance with legal and ethical standards in research and experimentation.