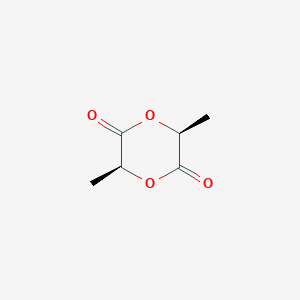
(3S,6S)-3,6-dimethyl-1,4-dioxane-2,5-dione
Overview
Description
Antrafenine Dihydrochloride is a phenylpiperazine derivative drug that was invented in 1979. It acts as an analgesic and anti-inflammatory agent with similar efficacy to naproxen. it is not widely used today as it has largely been replaced by newer drugs .
Mechanism of Action
L-Lactide, also known as L-(-)-Lactide, LL-Lactide, or (3S,6S)-3,6-dimethyl-1,4-dioxane-2,5-dione, is a cyclic ester used as an intermediate product in the production of poly(lactic acid) (PLA), a high-molar mass polymer .
Target of Action
L-Lactide’s primary target is the polymerization process that leads to the formation of PLA . The polymerization of L,L-lactide obtains a linear homopolymer chain architecture with high molar mass .
Mode of Action
L-Lactide interacts with its targets through a process known as ring-opening polymerization . This process involves the breaking of the cyclic ester bond in L-Lactide, allowing it to form linear chains that constitute PLA .
Biochemical Pathways
The biochemical pathway involved in the action of L-Lactide is the polymerization process. This process is initiated by the dehydration of lactic acid, followed by the depolymerization of the resulting prepolymer, and subsequent purification . The result is the formation of L-Lactide, which can then be polymerized to form PLA .
Pharmacokinetics
The pharmacokinetics of L-Lactide and its resulting PLA are determined by the properties of the PLA polymer. The ADME (Absorption, Distribution, Metabolism, and Excretion) properties of PLA are influenced by factors such as the molecular weight of the polymer and the presence of any copolymers . These factors can impact the bioavailability of drugs delivered using PLA-based systems .
Result of Action
The result of L-Lactide’s action is the formation of PLA, a biodegradable polymer with a wide range of applications. PLA is used in various fields, including medical applications (e.g., prostheses and membranes), drug delivery, and hydrogels, or combined with other polymers for applications in packaging .
Action Environment
The action of L-Lactide is influenced by environmental factors such as temperature and pressure. The synthesis of L-Lactide requires strict control of these factors, as well as the use of catalysts . Furthermore, the properties of the resulting PLA can be influenced by the presence of other substances, such as gallium nanoparticles, which can enhance the antibacterial properties of the PLA .
Biochemical Analysis
Biochemical Properties
L-Lactide plays a vital role in biochemical reactions, particularly in the synthesis of polylactic acid (PLA). It interacts with various enzymes, proteins, and other biomolecules during its polymerization process. One of the key enzymes involved is lactate dehydrogenase, which catalyzes the conversion of lactic acid to L-Lactide . Additionally, L-Lactide interacts with catalysts such as tin octoate during the ring-opening polymerization process to form PLA . These interactions are crucial for the efficient production of high-quality PLA with desirable properties.
Cellular Effects
L-Lactide influences various cellular processes, including cell signaling pathways, gene expression, and cellular metabolism. Studies have shown that L-Lactide-based materials, such as PLA, can affect cell proliferation and differentiation . For instance, PLA scaffolds have been used in tissue engineering to support the growth and differentiation of stem cells into specific cell types . Additionally, L-Lactide can impact cellular metabolism by providing a biodegradable matrix that cells can interact with, promoting tissue regeneration and repair .
Molecular Mechanism
The molecular mechanism of L-Lactide involves its polymerization into polylactic acid (PLA) through ring-opening polymerization. During this process, L-Lactide undergoes a series of chemical reactions catalyzed by tin octoate, resulting in the formation of long PLA chains . These PLA chains can then interact with various biomolecules, influencing cellular functions and gene expression. For example, PLA scaffolds can provide structural support for cells, promoting their attachment, proliferation, and differentiation . Additionally, the degradation of PLA releases lactic acid, which can further influence cellular metabolism and gene expression .
Temporal Effects in Laboratory Settings
In laboratory settings, the effects of L-Lactide can change over time due to its stability and degradation properties. L-Lactide is known to be relatively stable under controlled conditions, but it can degrade over time, especially in the presence of moisture and heat . This degradation can lead to changes in the mechanical properties of PLA-based materials, affecting their performance in various applications. Long-term studies have shown that the degradation of PLA can result in the release of lactic acid, which can influence cellular functions and tissue regeneration .
Dosage Effects in Animal Models
The effects of L-Lactide can vary with different dosages in animal models. Studies have shown that low doses of L-Lactide-based materials, such as PLA, are generally well-tolerated and can promote tissue regeneration and repair . High doses of L-Lactide or PLA can lead to adverse effects, such as inflammation and tissue damage . It is essential to determine the optimal dosage of L-Lactide-based materials to achieve the desired therapeutic effects while minimizing potential toxicity.
Metabolic Pathways
L-Lactide is involved in various metabolic pathways, primarily through its conversion to lactic acid. The enzyme lactate dehydrogenase catalyzes the conversion of lactic acid to L-Lactide, which can then be polymerized into PLA . Additionally, the degradation of PLA releases lactic acid, which can enter various metabolic pathways, including glycolysis and the tricarboxylic acid (TCA) cycle . These metabolic pathways are crucial for cellular energy production and overall metabolic homeostasis.
Transport and Distribution
L-Lactide is transported and distributed within cells and tissues through various mechanisms. It can interact with transporters and binding proteins that facilitate its uptake and distribution . Once inside the cells, L-Lactide can be incorporated into PLA-based materials, providing structural support for tissue regeneration and repair . Additionally, the degradation of PLA releases lactic acid, which can be transported to other tissues and organs, influencing their metabolic functions .
Subcellular Localization
The subcellular localization of L-Lactide and its derivatives, such as PLA, can influence their activity and function. L-Lactide-based materials can be targeted to specific cellular compartments or organelles through various mechanisms, including post-translational modifications and targeting signals . For example, PLA nanoparticles can be designed to target specific cellular compartments, such as the cytoplasm or nucleus, to deliver therapeutic agents or support tissue regeneration . The localization of L-Lactide and PLA within cells can significantly impact their biological activity and therapeutic potential.
Preparation Methods
Synthetic Routes and Reaction Conditions: The synthesis of Antrafenine involves multiple steps. One of the methods includes the reaction between 2-[4-[3-(trifluoromethyl)phenyl]-1-piperazinyl]ethanol and Isatoic anhydride to form 4-(3-(Trifluoromethyl)phenyl)piperazine-1-ethyl 2-aminobenzoate. This intermediate is then alkylated with 4-chloro-7-(trifluoromethyl)quinoline to complete the synthesis of Antrafenine .
Industrial Production Methods: Industrial production methods for Antrafenine Dihydrochloride are not widely documented, but they likely follow similar synthetic routes with optimizations for large-scale production, including the use of automated reactors and purification systems to ensure high yield and purity.
Chemical Reactions Analysis
Types of Reactions: Antrafenine Dihydrochloride undergoes various chemical reactions, including:
Oxidation: It can be oxidized under specific conditions to form different derivatives.
Reduction: Reduction reactions can modify its functional groups.
Substitution: It can undergo substitution reactions, particularly involving its phenyl and piperazine rings.
Common Reagents and Conditions: Common reagents used in these reactions include oxidizing agents like potassium permanganate, reducing agents like lithium aluminum hydride, and various halogenating agents for substitution reactions.
Major Products: The major products formed from these reactions depend on the specific conditions and reagents used. For example, oxidation may yield quinoline derivatives, while substitution reactions can introduce various functional groups into the phenyl or piperazine rings .
Scientific Research Applications
Antrafenine Dihydrochloride has been studied for its anti-inflammatory and analgesic properties. Additionally, it has been explored for its potential in drug metabolism studies and the analysis of impurities and degradation products in pharmaceuticals .
Comparison with Similar Compounds
Naproxen: Antrafenine Dihydrochloride has similar efficacy to naproxen as an analgesic and anti-inflammatory agent.
Phenylbutazone: It has been compared to phenylbutazone in terms of its anti-inflammatory effects.
Uniqueness: Antrafenine Dihydrochloride is unique in its specific chemical structure, which includes a trifluoromethyl group on the phenyl ring and a piperazine ring. This structure contributes to its specific pharmacological properties and distinguishes it from other similar compounds .
Properties
IUPAC Name |
(3S,6S)-3,6-dimethyl-1,4-dioxane-2,5-dione | |
---|---|---|
Source | PubChem | |
URL | https://pubchem.ncbi.nlm.nih.gov | |
Description | Data deposited in or computed by PubChem | |
InChI |
InChI=1S/C6H8O4/c1-3-5(7)10-4(2)6(8)9-3/h3-4H,1-2H3/t3-,4-/m0/s1 | |
Source | PubChem | |
URL | https://pubchem.ncbi.nlm.nih.gov | |
Description | Data deposited in or computed by PubChem | |
InChI Key |
JJTUDXZGHPGLLC-IMJSIDKUSA-N | |
Source | PubChem | |
URL | https://pubchem.ncbi.nlm.nih.gov | |
Description | Data deposited in or computed by PubChem | |
Canonical SMILES |
CC1C(=O)OC(C(=O)O1)C | |
Source | PubChem | |
URL | https://pubchem.ncbi.nlm.nih.gov | |
Description | Data deposited in or computed by PubChem | |
Isomeric SMILES |
C[C@H]1C(=O)O[C@H](C(=O)O1)C | |
Source | PubChem | |
URL | https://pubchem.ncbi.nlm.nih.gov | |
Description | Data deposited in or computed by PubChem | |
Molecular Formula |
C6H8O4 | |
Source | PubChem | |
URL | https://pubchem.ncbi.nlm.nih.gov | |
Description | Data deposited in or computed by PubChem | |
Related CAS |
33135-50-1 | |
Record name | L-Lactide homopolymer | |
Source | CAS Common Chemistry | |
URL | https://commonchemistry.cas.org/detail?cas_rn=33135-50-1 | |
Description | CAS Common Chemistry is an open community resource for accessing chemical information. Nearly 500,000 chemical substances from CAS REGISTRY cover areas of community interest, including common and frequently regulated chemicals, and those relevant to high school and undergraduate chemistry classes. This chemical information, curated by our expert scientists, is provided in alignment with our mission as a division of the American Chemical Society. | |
Explanation | The data from CAS Common Chemistry is provided under a CC-BY-NC 4.0 license, unless otherwise stated. | |
DSSTOX Substance ID |
DTXSID5049655 | |
Record name | L-Dilactide | |
Source | EPA DSSTox | |
URL | https://comptox.epa.gov/dashboard/DTXSID5049655 | |
Description | DSSTox provides a high quality public chemistry resource for supporting improved predictive toxicology. | |
Molecular Weight |
144.12 g/mol | |
Source | PubChem | |
URL | https://pubchem.ncbi.nlm.nih.gov | |
Description | Data deposited in or computed by PubChem | |
CAS No. |
4511-42-6, 33135-50-1 | |
Record name | L-Lactide | |
Source | CAS Common Chemistry | |
URL | https://commonchemistry.cas.org/detail?cas_rn=4511-42-6 | |
Description | CAS Common Chemistry is an open community resource for accessing chemical information. Nearly 500,000 chemical substances from CAS REGISTRY cover areas of community interest, including common and frequently regulated chemicals, and those relevant to high school and undergraduate chemistry classes. This chemical information, curated by our expert scientists, is provided in alignment with our mission as a division of the American Chemical Society. | |
Explanation | The data from CAS Common Chemistry is provided under a CC-BY-NC 4.0 license, unless otherwise stated. | |
Record name | Lactide, L- | |
Source | ChemIDplus | |
URL | https://pubchem.ncbi.nlm.nih.gov/substance/?source=chemidplus&sourceid=0004511426 | |
Description | ChemIDplus is a free, web search system that provides access to the structure and nomenclature authority files used for the identification of chemical substances cited in National Library of Medicine (NLM) databases, including the TOXNET system. | |
Record name | 1,4-Dioxane-2,5-dione, 3,6-dimethyl-, (3S-cis)-, homopolymer | |
Source | ChemIDplus | |
URL | https://pubchem.ncbi.nlm.nih.gov/substance/?source=chemidplus&sourceid=0033135501 | |
Description | ChemIDplus is a free, web search system that provides access to the structure and nomenclature authority files used for the identification of chemical substances cited in National Library of Medicine (NLM) databases, including the TOXNET system. | |
Record name | 1,4-Dioxane-2,5-dione, 3,6-dimethyl-, (3S,6S)- | |
Source | EPA Chemicals under the TSCA | |
URL | https://www.epa.gov/chemicals-under-tsca | |
Description | EPA Chemicals under the Toxic Substances Control Act (TSCA) collection contains information on chemicals and their regulations under TSCA, including non-confidential content from the TSCA Chemical Substance Inventory and Chemical Data Reporting. | |
Record name | L-Dilactide | |
Source | EPA DSSTox | |
URL | https://comptox.epa.gov/dashboard/DTXSID5049655 | |
Description | DSSTox provides a high quality public chemistry resource for supporting improved predictive toxicology. | |
Record name | (3S-cis)-3,6-dimethyl-1,4-dioxane-2,5-dione | |
Source | European Chemicals Agency (ECHA) | |
URL | https://echa.europa.eu/substance-information/-/substanceinfo/100.022.576 | |
Description | The European Chemicals Agency (ECHA) is an agency of the European Union which is the driving force among regulatory authorities in implementing the EU's groundbreaking chemicals legislation for the benefit of human health and the environment as well as for innovation and competitiveness. | |
Explanation | Use of the information, documents and data from the ECHA website is subject to the terms and conditions of this Legal Notice, and subject to other binding limitations provided for under applicable law, the information, documents and data made available on the ECHA website may be reproduced, distributed and/or used, totally or in part, for non-commercial purposes provided that ECHA is acknowledged as the source: "Source: European Chemicals Agency, http://echa.europa.eu/". Such acknowledgement must be included in each copy of the material. ECHA permits and encourages organisations and individuals to create links to the ECHA website under the following cumulative conditions: Links can only be made to webpages that provide a link to the Legal Notice page. | |
Record name | LACTIDE, L- | |
Source | FDA Global Substance Registration System (GSRS) | |
URL | https://gsrs.ncats.nih.gov/ginas/app/beta/substances/IJ13TO4NO1 | |
Description | The FDA Global Substance Registration System (GSRS) enables the efficient and accurate exchange of information on what substances are in regulated products. Instead of relying on names, which vary across regulatory domains, countries, and regions, the GSRS knowledge base makes it possible for substances to be defined by standardized, scientific descriptions. | |
Explanation | Unless otherwise noted, the contents of the FDA website (www.fda.gov), both text and graphics, are not copyrighted. They are in the public domain and may be republished, reprinted and otherwise used freely by anyone without the need to obtain permission from FDA. Credit to the U.S. Food and Drug Administration as the source is appreciated but not required. | |
Synthesis routes and methods I
Procedure details
Synthesis routes and methods II
Procedure details
Retrosynthesis Analysis
AI-Powered Synthesis Planning: Our tool employs the Template_relevance Pistachio, Template_relevance Bkms_metabolic, Template_relevance Pistachio_ringbreaker, Template_relevance Reaxys, Template_relevance Reaxys_biocatalysis model, leveraging a vast database of chemical reactions to predict feasible synthetic routes.
One-Step Synthesis Focus: Specifically designed for one-step synthesis, it provides concise and direct routes for your target compounds, streamlining the synthesis process.
Accurate Predictions: Utilizing the extensive PISTACHIO, BKMS_METABOLIC, PISTACHIO_RINGBREAKER, REAXYS, REAXYS_BIOCATALYSIS database, our tool offers high-accuracy predictions, reflecting the latest in chemical research and data.
Strategy Settings
Precursor scoring | Relevance Heuristic |
---|---|
Min. plausibility | 0.01 |
Model | Template_relevance |
Template Set | Pistachio/Bkms_metabolic/Pistachio_ringbreaker/Reaxys/Reaxys_biocatalysis |
Top-N result to add to graph | 6 |
Feasible Synthetic Routes
Disclaimer and Information on In-Vitro Research Products
Please be aware that all articles and product information presented on BenchChem are intended solely for informational purposes. The products available for purchase on BenchChem are specifically designed for in-vitro studies, which are conducted outside of living organisms. In-vitro studies, derived from the Latin term "in glass," involve experiments performed in controlled laboratory settings using cells or tissues. It is important to note that these products are not categorized as medicines or drugs, and they have not received approval from the FDA for the prevention, treatment, or cure of any medical condition, ailment, or disease. We must emphasize that any form of bodily introduction of these products into humans or animals is strictly prohibited by law. It is essential to adhere to these guidelines to ensure compliance with legal and ethical standards in research and experimentation.