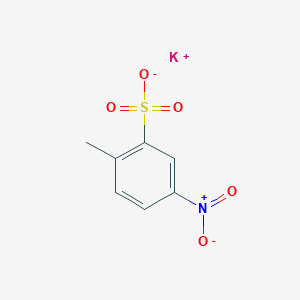
Potassium 2-methyl-5-nitrobenzenesulfonate
- Click on QUICK INQUIRY to receive a quote from our team of experts.
- With the quality product at a COMPETITIVE price, you can focus more on your research.
Overview
Description
Potassium 2-methyl-5-nitrobenzenesulfonate is an organic compound with the molecular formula K+·C7H6NO5S−. It consists of a two-dimensional framework of potassium ions coordinated to 2-methyl-5-nitrobenzenesulfonate anions. The potassium ion is typically eight-coordinate, surrounded by eight oxygen atoms from six 2-methyl-5-nitrobenzenesulfonate anions .
Preparation Methods
Synthetic Routes and Reaction Conditions
The synthesis of potassium 2-methyl-5-nitrobenzenesulfonate typically involves the nitration of 2-methylbenzenesulfonic acid followed by neutralization with potassium hydroxide. The nitration reaction is carried out using a mixture of concentrated sulfuric acid and nitric acid at a controlled temperature to introduce the nitro group at the 5-position of the benzene ring. The resulting 2-methyl-5-nitrobenzenesulfonic acid is then neutralized with potassium hydroxide to form the potassium salt .
Industrial Production Methods
Industrial production of this compound follows similar synthetic routes but on a larger scale. The reaction conditions are optimized for higher yields and purity. The process involves continuous monitoring of temperature and pH levels to ensure the complete conversion of reactants to the desired product. The final product is purified through recrystallization or other suitable methods to achieve the required quality standards .
Chemical Reactions Analysis
Types of Reactions
Potassium 2-methyl-5-nitrobenzenesulfonate undergoes various chemical reactions, including:
Oxidation: The nitro group can be reduced to an amino group under suitable conditions.
Reduction: The compound can be reduced using reducing agents like hydrogen gas in the presence of a catalyst.
Substitution: The sulfonate group can participate in nucleophilic substitution reactions
Common Reagents and Conditions
Oxidation: Common oxidizing agents include potassium permanganate and chromium trioxide.
Reduction: Reducing agents such as hydrogen gas with a palladium catalyst or sodium borohydride are used.
Substitution: Nucleophiles like amines or alcohols can react with the sulfonate group under basic conditions
Major Products Formed
Oxidation: 2-methyl-5-nitrobenzenesulfonic acid.
Reduction: 2-methyl-5-aminobenzenesulfonate.
Substitution: Various substituted benzenesulfonates depending on the nucleophile used
Scientific Research Applications
Potassium 2-methyl-5-nitrobenzenesulfonate is significant as an intermediate in the production of dyes, pigments, and agricultural chemicals . Its role in synthesizing various active compounds for use in pharmaceutics, crop protection, polymers, and dyes highlights its importance in these technical areas .
Production and Use
- Synthesis : this compound can be produced through the sulfonation of p-nitrotoluene with SO3 gas, followed by neutralization with a potassium base .
- Intermediate for Active Compounds : 2-Carboxy-5-nitrobenzenesulfonic acid, derived from this compound, is utilized in the synthesis of active pharmaceutical and agricultural compounds .
- Agricultural Applications : It is used in the development of agricultural chemicals that control weed growth .
Chemical Processes and Applications
The process for preparing 2-carboxy-5-nitrobenzenesulfonic acid, which involves the oxidation of 2-methyl-5-nitrobenzenesulfonic acid, demonstrates the utility of this compound in creating other important chemical intermediates . The oxidation can be performed using metal hypochlorites in the presence of metal bases, showcasing a specific application in chemical synthesis .
Additional Applications
This compound is also used in the production of:
Mechanism of Action
The mechanism of action of potassium 2-methyl-5-nitrobenzenesulfonate involves its interaction with specific molecular targets. The nitro group can undergo reduction to form reactive intermediates that interact with cellular components. These interactions can lead to the inhibition of enzyme activity or disruption of cellular processes. The sulfonate group can also participate in binding interactions with proteins and other biomolecules, affecting their function .
Comparison with Similar Compounds
Similar Compounds
- 2-methyl-5-nitrobenzenesulfonic acid
2-methyl-5-nitrobenzenesulfonamide: Similar structure but with an amide group instead of a potassium salt.
Biological Activity
Potassium 2-methyl-5-nitrobenzenesulfonate (K⁺·C₇H₆NO₅S⁻) is a compound that has garnered interest due to its diverse biological activities. This article explores the biological mechanisms, potential therapeutic applications, and relevant research findings associated with this compound.
Chemical Structure and Properties
This compound features a nitro group which is known to impart various biological activities. The presence of the sulfonate group enhances its solubility in aqueous environments, making it suitable for biological applications. The molecular structure can be represented as follows:
Antimicrobial Properties
Research indicates that nitro compounds, including this compound, exhibit significant antimicrobial properties. Nitro groups are known to be critical for the activity against various pathogens. For instance, studies have shown that similar nitro compounds can effectively inhibit the growth of Mycobacterium tuberculosis, which is resistant to many traditional antibiotics. The mechanism often involves the reduction of the nitro group to form reactive nitrogen species that damage bacterial DNA and proteins .
Anti-inflammatory Effects
The compound also demonstrates anti-inflammatory properties, potentially through the inhibition of key inflammatory mediators such as nitric oxide synthase (iNOS) and cyclooxygenase-2 (COX-2). This action can lead to reduced production of pro-inflammatory cytokines like TNF-α and IL-1β, which are implicated in various inflammatory diseases . The ability of this compound to modulate these pathways suggests its potential use in treating inflammation-related conditions.
Vasodilatory Activity
Nitro compounds are recognized for their vasodilatory effects due to their ability to release nitric oxide (NO) upon bio-reduction. This process leads to increased levels of cyclic guanosine monophosphate (cGMP) in vascular smooth muscle cells, resulting in relaxation and dilation of blood vessels. Such properties make this compound a candidate for therapeutic use in cardiovascular diseases .
Synthesis and Characterization
The synthesis of this compound has been documented, showcasing methods that yield high purity and stability. Characterization techniques such as NMR and mass spectrometry confirm the structure and purity of the compound .
In Vitro Studies
In vitro studies have demonstrated the efficacy of this compound against various cell lines. For example, it has shown promising results in inhibiting cell proliferation in cancer models, indicating potential antineoplastic activity. The minimum inhibitory concentration (MIC) values for related nitro compounds have been reported as low as 0.78 μM against M. tuberculosis, suggesting that this compound may possess similar potency .
Data Table: Summary of Biological Activities
Properties
Molecular Formula |
C7H6KNO5S |
---|---|
Molecular Weight |
255.29 g/mol |
IUPAC Name |
potassium;2-methyl-5-nitrobenzenesulfonate |
InChI |
InChI=1S/C7H7NO5S.K/c1-5-2-3-6(8(9)10)4-7(5)14(11,12)13;/h2-4H,1H3,(H,11,12,13);/q;+1/p-1 |
InChI Key |
RPTBTSBTSYDGIO-UHFFFAOYSA-M |
Canonical SMILES |
CC1=C(C=C(C=C1)[N+](=O)[O-])S(=O)(=O)[O-].[K+] |
Origin of Product |
United States |
Disclaimer and Information on In-Vitro Research Products
Please be aware that all articles and product information presented on BenchChem are intended solely for informational purposes. The products available for purchase on BenchChem are specifically designed for in-vitro studies, which are conducted outside of living organisms. In-vitro studies, derived from the Latin term "in glass," involve experiments performed in controlled laboratory settings using cells or tissues. It is important to note that these products are not categorized as medicines or drugs, and they have not received approval from the FDA for the prevention, treatment, or cure of any medical condition, ailment, or disease. We must emphasize that any form of bodily introduction of these products into humans or animals is strictly prohibited by law. It is essential to adhere to these guidelines to ensure compliance with legal and ethical standards in research and experimentation.