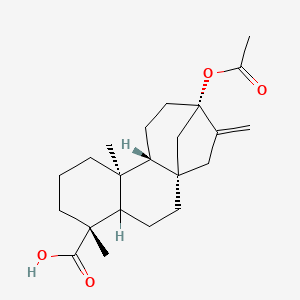
Steviol acetate
Overview
Description
Steviol acetate (C₂₂H₃₂O₄) is a semi-synthetic derivative of steviol, the aglycone core of steviol glycosides found in Stevia rebaudiana Bertoni. It is synthesized via acetylation of steviol, typically using acetic anhydride or acetyl chloride under alkaline conditions . Key structural features include a diterpenoid backbone (ent-kaurene skeleton) with an acetyl group at the C-13 hydroxyl position (Figure 1). Its molecular weight is 360.49 g/mol, and it exhibits distinct spectroscopic properties:
- ¹H-NMR (CDCl₃): δ = 2.060 (s, 3H, OCOCH₃), 1.292 (s, 3H, H-19), 1.001 (s, 3H, H-20) .
- ESI-MS: m/z 383.4 [M+Na]⁺ .
This compound serves as a precursor in the synthesis of bioactive derivatives, such as this compound ketone (C₂₁H₃₀O₅), through oxidation and methylenation reactions . Unlike steviol glycosides, it lacks sugar moieties, which alters its solubility and metabolic pathways.
Preparation Methods
Preparation Methods
Chemical Synthesis
The chemical synthesis of steviol acetate typically involves the acetylation of steviol, which can be derived from stevioside or rebaudioside A through hydrolysis. Below are the detailed steps involved in the chemical synthesis:
-
- The crude steviol is then treated with acetic anhydride in the presence of a base (such as pyridine) to facilitate acetylation.
- After the reaction, excess acetic anhydride is removed under reduced pressure, and the product is extracted with ether.
- The ether layer is washed, dried, and evaporated to yield crude this compound, which can be further purified through recrystallization.
Enzymatic Methods
Enzymatic synthesis offers a more environmentally friendly alternative to traditional chemical methods. This method typically employs enzymes such as pectinase to hydrolyze stevioside into steviol before acetylation:
-
- Stevioside is treated with crude pectinase at controlled temperatures (around 45 °C) in a phosphate buffer solution.
- Following hydrolysis, the resulting steviol precipitates out and is filtered.
Research Findings
Recent studies have highlighted various aspects of the preparation methods for this compound, including yield efficiency and purity levels:
A study reported that using sodium periodate for hydrolysis followed by acetylation yielded approximately 56% pure this compound after purification.
Another research indicated that enzymatic methods could provide a yield of about 25% for pure steviol from stevioside, showcasing the potential for biocatalysis in producing natural sweeteners.
Comparative Analysis of Preparation Methods
The following table summarizes key differences between chemical and enzymatic methods for preparing this compound:
Method | Yield (%) | Purification Steps | Environmental Impact |
---|---|---|---|
Chemical Synthesis | ~56 | Column chromatography | Moderate |
Enzymatic Method | ~25 | Minimal (filtration) | Low |
Chemical Reactions Analysis
Oxidation to Steviol Acetate Nor-Ketone
Controlled oxidation converts the C16–C17 double bond into a ketone:
-
Mechanism : Oxidative cleavage of the exocyclic methylene group forms a 17-nor-16-ketone derivative.
-
Impact : The ketone derivative loses sweet taste properties, confirming the C16–C17 double bond’s role as a pharmacophore .
Methylenation to Regenerate the Double Bond
The ketone is converted back to the methylene group via a novel methylenation protocol:
-
Outcome : Restoration of the double bond, confirmed by C NMR (δ 214–215 for ketone vs. δ 192 for regenerated this compound) .
Hydrolysis and Derivatization
This compound undergoes hydrolysis to regenerate steviol or form derivatives:
-
Base Hydrolysis : Refluxing with 2M KOH followed by acidification yields steviol (85% yield) .
-
Enzymatic Modification : Cyclomaltodextrin glucanotransferase introduces glucosyl units, altering solubility and stability .
Comparative Reaction Data
Reaction Type | Reagents/Conditions | Product | Yield |
---|---|---|---|
Acetylation | AcO, pyridine/DMAP | This compound | 56% |
Oxidation | OsO, NaIO, THF/HO | This compound nor-ketone | 71% |
Methylenation | Zn/CHBr/TiCl | Regenerated this compound | 65% |
Key Findings
-
Structural Sensitivity : The C16–C17 double bond is essential for sweetness; its modification (e.g., ketone formation) abolishes taste .
-
Spectral Signatures : C NMR distinguishes ketone (δ 214–215) and methylene (δ 192) derivatives .
-
Synthetic Utility : Acetylation and methylenation enable scalable production of steviol analogs for industrial applications .
Scientific Research Applications
Natural Sweetener
Sweetness Profile
Steviol acetate is significantly sweeter than sucrose, with a sweetness intensity estimated to be 200 to 300 times that of sucrose . This makes it an attractive alternative for sugar replacement in food products, especially for those seeking to reduce caloric intake.
Stability in Food Products
Research indicates that steviol glycosides, including this compound, exhibit remarkable stability under various pH conditions, making them suitable for use in acidic beverages. For instance, studies have shown minimal decomposition of steviol glycosides at pH levels typical for soft drinks over extended periods . This stability ensures that the sweetening properties remain intact during storage.
Health Benefits
Antioxidant Properties
This compound has demonstrated antioxidant effects in various studies. For example, extracts from Stevia rebaudiana, which contain steviol derivatives, have been shown to reduce oxidative stress markers in animal models. Specifically, stevioside and related compounds have been reported to decrease lipid peroxidation and enhance antioxidant enzyme activities in diabetic rats . These findings suggest potential therapeutic applications for this compound in managing oxidative stress-related conditions.
Antidiabetic Effects
This compound and its glycosides are being investigated for their antidiabetic properties. Studies indicate that these compounds may help regulate blood glucose levels by enhancing insulin sensitivity and reducing hyperglycemia . Given the rising prevalence of diabetes globally, the incorporation of this compound into dietary regimens could provide a natural approach to glycemic control.
Metabolic Studies
Biotransformation Research
Research into the metabolism of steviol and its derivatives has revealed insights into their biochemical pathways. For instance, studies using Gibberella fujikuroi have shown how steviol is metabolized in the presence of specific inhibitors . Understanding these metabolic processes is crucial for assessing the bioavailability and efficacy of this compound as a dietary supplement or therapeutic agent.
Case Studies
Study | Focus | Findings |
---|---|---|
Prata et al. (2017) | Antioxidant effects | Steviol derivatives reduced oxidative stress markers in vitro. |
Rotimi et al. (2018) | Antidiabetic potential | Stevioside significantly lowered blood glucose levels in diabetic rats. |
Ghanta et al. (2007) | DNA protection | Ethyl acetate extracts prevented DNA breaks caused by free radicals. |
Mechanism of Action
The mechanism of action of steviol acetate involves its interaction with various molecular targets and pathways. For instance, this compound has been shown to inhibit DNA polymerases and human DNA topoisomerase II, which are critical enzymes involved in DNA replication and cell division. This inhibition can lead to the suppression of cancer cell growth, making this compound a potential candidate for cancer therapy.
Comparison with Similar Compounds
Comparative Analysis with Structurally Related Compounds
Structural and Functional Group Comparisons
Key Observations:
- Sugar Moieties: Steviol glycosides (stevioside, rebaudioside A) are water-soluble due to glycosylation, whereas this compound and isosteviol are lipophilic .
- Bioactivity Modulation: The acetyl group in this compound enhances its stability in organic solvents, making it suitable for synthetic modifications . In contrast, glycosides like rebaudioside A are metabolized to steviol in the gut, which interacts with gut microbiota to modulate short-chain fatty acids (e.g., acetate, butyrate) .
Metabolism
Q & A
Basic Research Questions
Q. What are the key steps in the chemical synthesis of steviol acetate from steviol?
this compound is synthesized via acetylation of steviol. The reaction involves steviol dissolved in acetic anhydride with dimethylaminopyridine (DMAP) as a catalyst. The mixture is stirred at room temperature for 48 hours, followed by purification steps to isolate this compound. This method ensures high regioselectivity for the C-19 hydroxyl group of steviol .
Q. How can enzymatic hydrolysis and UPLC-MS/MS be applied to quantify this compound metabolites in biological samples?
Plasma samples containing steviol glucuronide (a metabolite of this compound) are treated with β-glucuronidase (from Helix pomatia) in ammonium acetate buffer (pH 5) at 37°C for 6 hours. This cleaves glucuronic acid, releasing free steviol. Post-hydrolysis, methyl tert-butyl ether extraction and UPLC-MS/MS analysis enable quantification of total steviol. Subtracting free steviol (measured without hydrolysis) yields steviol glucuronide concentration, a critical step in pharmacokinetic studies .
Q. What analytical challenges arise in separating this compound from structurally similar compounds?
this compound’s polarity and structural similarity to other steviol derivatives (e.g., steviol glycosides) complicate separation. Mixed-mode reverse-phase weak anion-exchange chromatography (RP-WAX) with UV detection is effective. Validation parameters (linearity, LOD, LOQ) must be established to ensure specificity and accuracy, especially in complex matrices like plant extracts or biological fluids .
Advanced Research Questions
Q. How do human gut microbiota influence the metabolic fate of this compound?
this compound is hydrolyzed to steviol in the colon by gut microbiota. In vitro fecal homogenate studies show near-complete conversion (>94%) of steviol glycosides to steviol within 48 hours. This parallels the metabolism of rebaudioside A, confirming shared degradation pathways. Researchers must account for inter-individual microbiota variations when interpreting in vivo pharmacokinetic data .
Q. What methodological approaches resolve contradictions in this compound’s mutagenicity data?
Discrepancies arise from variable material purity and metabolic activation conditions. In vitro mutagenicity assays (e.g., Ames test) should use this compound standards with ≥95% purity (verified via HPLC). Human liver microsome studies can identify mutagenic metabolites, while in vivo carcinogenicity assays in rodents (e.g., 2-year bioassays) provide complementary data. JECFA’s safety assessment framework (100-fold safety factor) mitigates these contradictions .
Q. How does β-glucuronidase inhibition affect this compound metabolite quantification?
β-glucuronidase inhibitors (e.g., steviol or isosteviol) in biological samples can artificially reduce free steviol levels. High-performance thin-layer chromatography (HPTLC) with enzyme inhibition profiling identifies interfering compounds. Dual analysis—with and without β-glucuronidase treatment—ensures accurate quantification of steviol glucuronide .
Q. What validation criteria are critical for internal standard (IS) methods in this compound analysis?
IS methods must demonstrate linearity (R² > 0.995), recovery (85–115%), and precision (RSD < 5%). Deuterated steviol-d₃ is ideal for LC-MS/MS due to co-elution with analytes. Validation should include matrix-matched calibration curves to account for ion suppression/enhancement in complex samples like plasma or plant extracts .
Q. Why do in vitro and in vivo pharmacokinetic models for this compound yield divergent results?
Differences stem from bioavailability factors (e.g., intestinal absorption efficiency, first-pass metabolism). In vitro Caco-2 cell models often overestimate absorption compared to in vivo rodent studies. Incorporating physiologically based pharmacokinetic (PBPK) modeling bridges these gaps by simulating enterohepatic recirculation and tissue distribution .
Properties
IUPAC Name |
(1R,5R,9S,10R,13S)-13-acetyloxy-5,9-dimethyl-14-methylidenetetracyclo[11.2.1.01,10.04,9]hexadecane-5-carboxylic acid | |
---|---|---|
Source | PubChem | |
URL | https://pubchem.ncbi.nlm.nih.gov | |
Description | Data deposited in or computed by PubChem | |
InChI |
InChI=1S/C22H32O4/c1-14-12-21-10-6-16-19(3,8-5-9-20(16,4)18(24)25)17(21)7-11-22(14,13-21)26-15(2)23/h16-17H,1,5-13H2,2-4H3,(H,24,25)/t16?,17-,19+,20+,21+,22-/m0/s1 | |
Source | PubChem | |
URL | https://pubchem.ncbi.nlm.nih.gov | |
Description | Data deposited in or computed by PubChem | |
InChI Key |
OWNAFQAMMJOLHX-BQCLRKHDSA-N | |
Source | PubChem | |
URL | https://pubchem.ncbi.nlm.nih.gov | |
Description | Data deposited in or computed by PubChem | |
Canonical SMILES |
CC(=O)OC12CCC3C4(CCCC(C4CCC3(C1)CC2=C)(C)C(=O)O)C | |
Source | PubChem | |
URL | https://pubchem.ncbi.nlm.nih.gov | |
Description | Data deposited in or computed by PubChem | |
Isomeric SMILES |
CC(=O)O[C@@]12CC[C@H]3[C@@]4(CCC[C@@](C4CC[C@]3(C1)CC2=C)(C)C(=O)O)C | |
Source | PubChem | |
URL | https://pubchem.ncbi.nlm.nih.gov | |
Description | Data deposited in or computed by PubChem | |
Molecular Formula |
C22H32O4 | |
Source | PubChem | |
URL | https://pubchem.ncbi.nlm.nih.gov | |
Description | Data deposited in or computed by PubChem | |
DSSTOX Substance ID |
DTXSID70965899 | |
Record name | 13-(Acetyloxy)kaur-16-en-18-oic acid | |
Source | EPA DSSTox | |
URL | https://comptox.epa.gov/dashboard/DTXSID70965899 | |
Description | DSSTox provides a high quality public chemistry resource for supporting improved predictive toxicology. | |
Molecular Weight |
360.5 g/mol | |
Source | PubChem | |
URL | https://pubchem.ncbi.nlm.nih.gov | |
Description | Data deposited in or computed by PubChem | |
CAS No. |
51576-10-4 | |
Record name | Steviol acetate | |
Source | ChemIDplus | |
URL | https://pubchem.ncbi.nlm.nih.gov/substance/?source=chemidplus&sourceid=0051576104 | |
Description | ChemIDplus is a free, web search system that provides access to the structure and nomenclature authority files used for the identification of chemical substances cited in National Library of Medicine (NLM) databases, including the TOXNET system. | |
Record name | 13-(Acetyloxy)kaur-16-en-18-oic acid | |
Source | EPA DSSTox | |
URL | https://comptox.epa.gov/dashboard/DTXSID70965899 | |
Description | DSSTox provides a high quality public chemistry resource for supporting improved predictive toxicology. | |
Retrosynthesis Analysis
AI-Powered Synthesis Planning: Our tool employs the Template_relevance Pistachio, Template_relevance Bkms_metabolic, Template_relevance Pistachio_ringbreaker, Template_relevance Reaxys, Template_relevance Reaxys_biocatalysis model, leveraging a vast database of chemical reactions to predict feasible synthetic routes.
One-Step Synthesis Focus: Specifically designed for one-step synthesis, it provides concise and direct routes for your target compounds, streamlining the synthesis process.
Accurate Predictions: Utilizing the extensive PISTACHIO, BKMS_METABOLIC, PISTACHIO_RINGBREAKER, REAXYS, REAXYS_BIOCATALYSIS database, our tool offers high-accuracy predictions, reflecting the latest in chemical research and data.
Strategy Settings
Precursor scoring | Relevance Heuristic |
---|---|
Min. plausibility | 0.01 |
Model | Template_relevance |
Template Set | Pistachio/Bkms_metabolic/Pistachio_ringbreaker/Reaxys/Reaxys_biocatalysis |
Top-N result to add to graph | 6 |
Feasible Synthetic Routes
Disclaimer and Information on In-Vitro Research Products
Please be aware that all articles and product information presented on BenchChem are intended solely for informational purposes. The products available for purchase on BenchChem are specifically designed for in-vitro studies, which are conducted outside of living organisms. In-vitro studies, derived from the Latin term "in glass," involve experiments performed in controlled laboratory settings using cells or tissues. It is important to note that these products are not categorized as medicines or drugs, and they have not received approval from the FDA for the prevention, treatment, or cure of any medical condition, ailment, or disease. We must emphasize that any form of bodily introduction of these products into humans or animals is strictly prohibited by law. It is essential to adhere to these guidelines to ensure compliance with legal and ethical standards in research and experimentation.