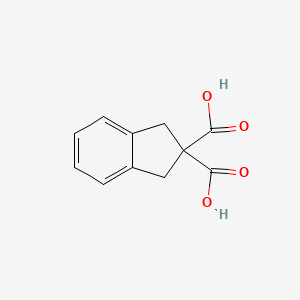
Indan-2,2-dicarboxylic acid
Overview
Description
Indan-2,2-dicarboxylic acid is an organic compound with the molecular formula C11H10O4. It is characterized by the presence of two carboxylic acid groups attached to the same carbon atom at position 2 of the indane ring. This compound is known for its unique bicyclic structure, which includes a benzene ring fused to a cyclopentane ring.
Preparation Methods
Synthetic Routes and Reaction Conditions: Indan-2,2-dicarboxylic acid can be synthesized through various methods. One common approach involves the oxidation of indan-2-carboxylic acid using strong oxidizing agents such as potassium permanganate or chromium trioxide. The reaction typically requires acidic conditions and elevated temperatures to proceed efficiently.
Industrial Production Methods: In industrial settings, the production of this compound often involves the catalytic oxidation of indane derivatives. This process can be carried out in large-scale reactors under controlled conditions to ensure high yield and purity of the final product.
Chemical Reactions Analysis
Types of Reactions: Indan-2,2-dicarboxylic acid undergoes various chemical reactions, including:
Oxidation: The compound can be further oxidized to form more complex derivatives.
Reduction: Reduction reactions can convert the carboxylic acid groups to alcohols or other functional groups.
Substitution: The aromatic ring of this compound can undergo electrophilic substitution reactions, such as nitration or halogenation.
Common Reagents and Conditions:
Oxidation: Potassium permanganate, chromium trioxide, and other strong oxidizing agents.
Reduction: Lithium aluminum hydride, sodium borohydride, and catalytic hydrogenation.
Substitution: Nitrating agents (e.g., nitric acid), halogenating agents (e.g., bromine, chlorine).
Major Products:
Oxidation: Formation of more oxidized derivatives.
Reduction: Conversion to alcohols or other reduced forms.
Substitution: Introduction of nitro, halogen, or other substituents on the aromatic ring.
Scientific Research Applications
Indan-2,2-dicarboxylic acid has a wide range of applications in scientific research:
Chemistry: Used as a building block for the synthesis of complex organic molecules and polymers.
Biology: Investigated for its potential biological activities and interactions with biomolecules.
Medicine: Explored for its potential therapeutic properties and as a precursor for drug development.
Industry: Utilized in the production of resins, coatings, and other industrial materials.
Mechanism of Action
The mechanism of action of indan-2,2-dicarboxylic acid involves its interaction with various molecular targets and pathways. The carboxylic acid groups can form hydrogen bonds and ionic interactions with proteins, enzymes, and other biomolecules, influencing their structure and function. Additionally, the aromatic ring can participate in π-π interactions and other non-covalent interactions, further modulating the compound’s effects.
Comparison with Similar Compounds
Indan-2,2-dicarboxylic acid can be compared with other dicarboxylic acids, such as:
Phthalic acid: Similar in having two carboxylic acid groups but differs in the position and structure of the aromatic ring.
Isophthalic acid: Also has two carboxylic acid groups but with a different arrangement on the benzene ring.
Terephthalic acid: Another dicarboxylic acid with carboxylic groups in the para position on the benzene ring.
Uniqueness: this compound is unique due to its bicyclic structure, which imparts distinct chemical and physical properties compared to other dicarboxylic acids. This structural uniqueness makes it valuable for specific applications in research and industry.
Biological Activity
Indan-2,2-dicarboxylic acid (IDCA) is an organic compound recognized for its unique bicyclic structure and potential biological activities. This article delves into its biological properties, mechanisms of action, and relevant research findings.
Chemical Structure and Properties
This compound has the molecular formula , featuring two carboxylic acid groups attached to the same carbon atom on the indane ring. This structure allows for various chemical reactions, including oxidation, reduction, and substitution, which can influence its biological activity.
The biological activity of IDCA is primarily attributed to its ability to interact with biomolecules. The carboxylic acid groups can form hydrogen bonds and ionic interactions with proteins and enzymes, potentially altering their structure and function. The aromatic ring facilitates π-π interactions , enhancing its binding capabilities with various biological targets.
Cytotoxicity
Research indicates that IDCA exhibits cytotoxic effects against certain cancer cell lines. For instance, studies have shown that compounds similar to IDCA can induce apoptosis in cancer cells through mechanisms involving cell cycle interference and oxidative stress. The cytotoxicity of dicarboxylic acids has been evaluated using various assays, including MTT assays, which measure cell viability in response to treatment .
Antioxidant Properties
IDCA and related compounds have demonstrated antioxidant activity. Antioxidants play a critical role in mitigating oxidative stress by scavenging free radicals, which are implicated in various diseases, including cancer. The incorporation of carboxylic acid functionalities has been shown to enhance the antioxidant capacity of related compounds .
Antimicrobial Activity
Dicarboxylic acids, including IDCA derivatives, have been studied for their antimicrobial properties. Coordination compounds formed with transition metals like copper and zinc have shown promising results against bacterial and fungal strains. These compounds' mechanisms often involve disrupting microbial cell membranes or inhibiting essential metabolic pathways .
Case Studies
- Cytotoxic Activity : In a study evaluating the cytotoxic effects of dicarboxylic acids on human cancer cell lines (HepG2 and MCF-7), it was found that certain derivatives exhibited significant cytotoxicity with IC50 values indicating selective toxicity towards cancerous cells compared to normal cells .
- Antioxidant Efficacy : Another research highlighted the enhanced antioxidant activity of IDCA derivatives when tested against standard antioxidants like vitamin C. The results indicated that these derivatives could effectively scavenge free radicals in vitro .
- Antimicrobial Properties : Coordination compounds of IDCA with transition metals were assessed for their antimicrobial efficacy. Results showed substantial inhibition of growth against various pathogens, suggesting potential applications in developing new antimicrobial agents .
Data Table: Summary of Biological Activities
Activity Type | Model System | Key Findings |
---|---|---|
Cytotoxicity | HepG2, MCF-7 | Significant IC50 values indicating selective toxicity |
Antioxidant | In vitro assays | Enhanced radical scavenging compared to vitamin C |
Antimicrobial | Various pathogens | Effective inhibition of microbial growth |
Properties
IUPAC Name |
1,3-dihydroindene-2,2-dicarboxylic acid | |
---|---|---|
Source | PubChem | |
URL | https://pubchem.ncbi.nlm.nih.gov | |
Description | Data deposited in or computed by PubChem | |
InChI |
InChI=1S/C11H10O4/c12-9(13)11(10(14)15)5-7-3-1-2-4-8(7)6-11/h1-4H,5-6H2,(H,12,13)(H,14,15) | |
Source | PubChem | |
URL | https://pubchem.ncbi.nlm.nih.gov | |
Description | Data deposited in or computed by PubChem | |
InChI Key |
RYGAENQWIQPFAI-UHFFFAOYSA-N | |
Source | PubChem | |
URL | https://pubchem.ncbi.nlm.nih.gov | |
Description | Data deposited in or computed by PubChem | |
Canonical SMILES |
C1C2=CC=CC=C2CC1(C(=O)O)C(=O)O | |
Source | PubChem | |
URL | https://pubchem.ncbi.nlm.nih.gov | |
Description | Data deposited in or computed by PubChem | |
Molecular Formula |
C11H10O4 | |
Source | PubChem | |
URL | https://pubchem.ncbi.nlm.nih.gov | |
Description | Data deposited in or computed by PubChem | |
DSSTOX Substance ID |
DTXSID60291078 | |
Record name | Indan-2,2-dicarboxylic acid | |
Source | EPA DSSTox | |
URL | https://comptox.epa.gov/dashboard/DTXSID60291078 | |
Description | DSSTox provides a high quality public chemistry resource for supporting improved predictive toxicology. | |
Molecular Weight |
206.19 g/mol | |
Source | PubChem | |
URL | https://pubchem.ncbi.nlm.nih.gov | |
Description | Data deposited in or computed by PubChem | |
CAS No. |
2437-08-3 | |
Record name | 2,2-Indandicarboxylic acid | |
Source | DTP/NCI | |
URL | https://dtp.cancer.gov/dtpstandard/servlet/dwindex?searchtype=NSC&outputformat=html&searchlist=73040 | |
Description | The NCI Development Therapeutics Program (DTP) provides services and resources to the academic and private-sector research communities worldwide to facilitate the discovery and development of new cancer therapeutic agents. | |
Explanation | Unless otherwise indicated, all text within NCI products is free of copyright and may be reused without our permission. Credit the National Cancer Institute as the source. | |
Record name | Indan-2,2-dicarboxylic acid | |
Source | EPA DSSTox | |
URL | https://comptox.epa.gov/dashboard/DTXSID60291078 | |
Description | DSSTox provides a high quality public chemistry resource for supporting improved predictive toxicology. | |
Record name | 1,3-dihydroindene-2,2-dicarboxylic acid | |
Source | European Chemicals Agency (ECHA) | |
URL | https://echa.europa.eu/information-on-chemicals | |
Description | The European Chemicals Agency (ECHA) is an agency of the European Union which is the driving force among regulatory authorities in implementing the EU's groundbreaking chemicals legislation for the benefit of human health and the environment as well as for innovation and competitiveness. | |
Explanation | Use of the information, documents and data from the ECHA website is subject to the terms and conditions of this Legal Notice, and subject to other binding limitations provided for under applicable law, the information, documents and data made available on the ECHA website may be reproduced, distributed and/or used, totally or in part, for non-commercial purposes provided that ECHA is acknowledged as the source: "Source: European Chemicals Agency, http://echa.europa.eu/". Such acknowledgement must be included in each copy of the material. ECHA permits and encourages organisations and individuals to create links to the ECHA website under the following cumulative conditions: Links can only be made to webpages that provide a link to the Legal Notice page. | |
Synthesis routes and methods I
Procedure details
Synthesis routes and methods II
Procedure details
Retrosynthesis Analysis
AI-Powered Synthesis Planning: Our tool employs the Template_relevance Pistachio, Template_relevance Bkms_metabolic, Template_relevance Pistachio_ringbreaker, Template_relevance Reaxys, Template_relevance Reaxys_biocatalysis model, leveraging a vast database of chemical reactions to predict feasible synthetic routes.
One-Step Synthesis Focus: Specifically designed for one-step synthesis, it provides concise and direct routes for your target compounds, streamlining the synthesis process.
Accurate Predictions: Utilizing the extensive PISTACHIO, BKMS_METABOLIC, PISTACHIO_RINGBREAKER, REAXYS, REAXYS_BIOCATALYSIS database, our tool offers high-accuracy predictions, reflecting the latest in chemical research and data.
Strategy Settings
Precursor scoring | Relevance Heuristic |
---|---|
Min. plausibility | 0.01 |
Model | Template_relevance |
Template Set | Pistachio/Bkms_metabolic/Pistachio_ringbreaker/Reaxys/Reaxys_biocatalysis |
Top-N result to add to graph | 6 |
Feasible Synthetic Routes
Q1: What is the spatial arrangement of the carboxyl groups in Indan-2,2-dicarboxylic acid?
A1: The two carboxyl groups in this compound adopt a synperiplanar conformation. This means they are oriented on the same side of the molecule, nearly eclipsing each other. This conformation is evident from the C-C-C-O torsion angles of 4.5 (3)° and -20.3 (3)° observed in the crystal structure. []
Q2: How does the structure of the cyclopentene ring in this compound deviate from planarity?
A2: The cyclopentene ring in this compound adopts an envelope conformation. This means that four of the five atoms in the ring lie in a plane, while the fifth atom (the one carrying the carboxyl group) deviates from this plane. Specifically, this carbon atom is located 0.355 (2) Å out of the plane defined by the other four carbon atoms in the ring. []
Disclaimer and Information on In-Vitro Research Products
Please be aware that all articles and product information presented on BenchChem are intended solely for informational purposes. The products available for purchase on BenchChem are specifically designed for in-vitro studies, which are conducted outside of living organisms. In-vitro studies, derived from the Latin term "in glass," involve experiments performed in controlled laboratory settings using cells or tissues. It is important to note that these products are not categorized as medicines or drugs, and they have not received approval from the FDA for the prevention, treatment, or cure of any medical condition, ailment, or disease. We must emphasize that any form of bodily introduction of these products into humans or animals is strictly prohibited by law. It is essential to adhere to these guidelines to ensure compliance with legal and ethical standards in research and experimentation.