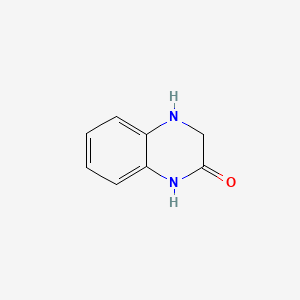
3,4-Dihydro-1H-quinoxalin-2-one
Overview
Description
3,4-Dihydro-1H-quinoxalin-2-one is an organic compound with the molecular formula C(_8)H(_8)N(_2)O. It is a derivative of quinoxaline, characterized by a bicyclic structure consisting of a benzene ring fused to a pyrazine ring. This compound is of significant interest due to its diverse applications in scientific research, particularly in the fields of chemistry, biology, and medicine.
Preparation Methods
Synthetic Routes and Reaction Conditions: The synthesis of 3,4-Dihydro-1H-quinoxalin-2-one typically involves the cyclization of o-phenylenediamine with α-keto acids or their derivatives. One common method includes the reaction of o-phenylenediamine with glyoxylic acid under acidic conditions, leading to the formation of the quinoxalinone ring.
Industrial Production Methods: Industrial production of this compound often employs similar synthetic routes but on a larger scale. The process involves careful control of reaction conditions such as temperature, pH, and solvent choice to ensure high yield and purity. The use of catalysts and optimized reaction parameters can enhance the efficiency of the synthesis.
Chemical Reactions Analysis
Types of Reactions: 3,4-Dihydro-1H-quinoxalin-2-one undergoes various chemical reactions, including:
Oxidation: It can be oxidized to form quinoxaline-2,3-dione.
Reduction: Reduction reactions can convert it to 3,4-dihydroquinoxaline.
Substitution: Electrophilic and nucleophilic substitution reactions can occur on the aromatic ring or the nitrogen atoms.
Common Reagents and Conditions:
Oxidation: Common oxidizing agents include potassium permanganate (KMnO(_4)) and hydrogen peroxide (H(_2)O(_2)).
Reduction: Reducing agents such as sodium borohydride (NaBH(_4)) or lithium aluminum hydride (LiAlH(_4)) are used.
Substitution: Reagents like halogens, alkyl halides, and acyl chlorides are employed under various conditions.
Major Products:
Oxidation: Quinoxaline-2,3-dione.
Reduction: 3,4-Dihydroquinoxaline.
Substitution: Various substituted quinoxalinones depending on the reagents used.
Scientific Research Applications
3,4-Dihydro-1H-quinoxalin-2-one has a wide range of applications in scientific research:
Chemistry: It serves as a building block for the synthesis of more complex molecules and as a ligand in coordination chemistry.
Biology: It is used in the study of enzyme inhibitors and as a scaffold for the development of bioactive compounds.
Medicine: Research has explored its potential as an antimicrobial, antiviral, and anticancer agent.
Industry: It is utilized in the development of dyes, pigments, and other industrial chemicals.
Mechanism of Action
The mechanism of action of 3,4-Dihydro-1H-quinoxalin-2-one varies depending on its application. In biological systems, it often interacts with enzymes or receptors, inhibiting their activity or modulating their function. The compound can bind to active sites or allosteric sites, altering the conformation and activity of the target molecules. Pathways involved may include inhibition of DNA synthesis, disruption of cell membrane integrity, or interference with metabolic processes.
Comparison with Similar Compounds
Quinoxaline: The parent compound, lacking the dihydro and oxo groups.
Quinoxaline-2,3-dione: An oxidized form of 3,4-Dihydro-1H-quinoxalin-2-one.
3,4-Dihydroquinoxaline: A reduced form of the compound.
Comparison: this compound is unique due to its specific structural features, which confer distinct chemical reactivity and biological activity. Compared to quinoxaline, it has additional functional groups that allow for more diverse chemical modifications and interactions. Its dihydro form provides different electronic properties, making it suitable for specific applications that quinoxaline or its other derivatives may not fulfill.
Properties
IUPAC Name |
3,4-dihydro-1H-quinoxalin-2-one | |
---|---|---|
Source | PubChem | |
URL | https://pubchem.ncbi.nlm.nih.gov | |
Description | Data deposited in or computed by PubChem | |
InChI |
InChI=1S/C8H8N2O/c11-8-5-9-6-3-1-2-4-7(6)10-8/h1-4,9H,5H2,(H,10,11) | |
Source | PubChem | |
URL | https://pubchem.ncbi.nlm.nih.gov | |
Description | Data deposited in or computed by PubChem | |
InChI Key |
HYTIPJFUWHYQON-UHFFFAOYSA-N | |
Source | PubChem | |
URL | https://pubchem.ncbi.nlm.nih.gov | |
Description | Data deposited in or computed by PubChem | |
Canonical SMILES |
C1C(=O)NC2=CC=CC=C2N1 | |
Source | PubChem | |
URL | https://pubchem.ncbi.nlm.nih.gov | |
Description | Data deposited in or computed by PubChem | |
Molecular Formula |
C8H8N2O | |
Source | PubChem | |
URL | https://pubchem.ncbi.nlm.nih.gov | |
Description | Data deposited in or computed by PubChem | |
DSSTOX Substance ID |
DTXSID50208236 | |
Record name | 2(1H)-Quinoxalinone, 3,4-dihydro- | |
Source | EPA DSSTox | |
URL | https://comptox.epa.gov/dashboard/DTXSID50208236 | |
Description | DSSTox provides a high quality public chemistry resource for supporting improved predictive toxicology. | |
Molecular Weight |
148.16 g/mol | |
Source | PubChem | |
URL | https://pubchem.ncbi.nlm.nih.gov | |
Description | Data deposited in or computed by PubChem | |
CAS No. |
59564-59-9 | |
Record name | 2(1H)-Quinoxalinone, 3,4-dihydro- | |
Source | ChemIDplus | |
URL | https://pubchem.ncbi.nlm.nih.gov/substance/?source=chemidplus&sourceid=0059564599 | |
Description | ChemIDplus is a free, web search system that provides access to the structure and nomenclature authority files used for the identification of chemical substances cited in National Library of Medicine (NLM) databases, including the TOXNET system. | |
Record name | 59564-59-9 | |
Source | DTP/NCI | |
URL | https://dtp.cancer.gov/dtpstandard/servlet/dwindex?searchtype=NSC&outputformat=html&searchlist=73534 | |
Description | The NCI Development Therapeutics Program (DTP) provides services and resources to the academic and private-sector research communities worldwide to facilitate the discovery and development of new cancer therapeutic agents. | |
Explanation | Unless otherwise indicated, all text within NCI products is free of copyright and may be reused without our permission. Credit the National Cancer Institute as the source. | |
Record name | 2(1H)-Quinoxalinone, 3,4-dihydro- | |
Source | EPA DSSTox | |
URL | https://comptox.epa.gov/dashboard/DTXSID50208236 | |
Description | DSSTox provides a high quality public chemistry resource for supporting improved predictive toxicology. | |
Record name | 3,4-DIHYDRO-2(1H)-QUINOXALINONE | |
Source | European Chemicals Agency (ECHA) | |
URL | https://echa.europa.eu/information-on-chemicals | |
Description | The European Chemicals Agency (ECHA) is an agency of the European Union which is the driving force among regulatory authorities in implementing the EU's groundbreaking chemicals legislation for the benefit of human health and the environment as well as for innovation and competitiveness. | |
Explanation | Use of the information, documents and data from the ECHA website is subject to the terms and conditions of this Legal Notice, and subject to other binding limitations provided for under applicable law, the information, documents and data made available on the ECHA website may be reproduced, distributed and/or used, totally or in part, for non-commercial purposes provided that ECHA is acknowledged as the source: "Source: European Chemicals Agency, http://echa.europa.eu/". Such acknowledgement must be included in each copy of the material. ECHA permits and encourages organisations and individuals to create links to the ECHA website under the following cumulative conditions: Links can only be made to webpages that provide a link to the Legal Notice page. | |
Synthesis routes and methods I
Procedure details
Synthesis routes and methods II
Procedure details
Synthesis routes and methods III
Procedure details
Synthesis routes and methods IV
Procedure details
Synthesis routes and methods V
Procedure details
Retrosynthesis Analysis
AI-Powered Synthesis Planning: Our tool employs the Template_relevance Pistachio, Template_relevance Bkms_metabolic, Template_relevance Pistachio_ringbreaker, Template_relevance Reaxys, Template_relevance Reaxys_biocatalysis model, leveraging a vast database of chemical reactions to predict feasible synthetic routes.
One-Step Synthesis Focus: Specifically designed for one-step synthesis, it provides concise and direct routes for your target compounds, streamlining the synthesis process.
Accurate Predictions: Utilizing the extensive PISTACHIO, BKMS_METABOLIC, PISTACHIO_RINGBREAKER, REAXYS, REAXYS_BIOCATALYSIS database, our tool offers high-accuracy predictions, reflecting the latest in chemical research and data.
Strategy Settings
Precursor scoring | Relevance Heuristic |
---|---|
Min. plausibility | 0.01 |
Model | Template_relevance |
Template Set | Pistachio/Bkms_metabolic/Pistachio_ringbreaker/Reaxys/Reaxys_biocatalysis |
Top-N result to add to graph | 6 |
Feasible Synthetic Routes
Q1: What are the common synthetic approaches to 3,4-Dihydro-1H-quinoxalin-2-ones?
A1: Several methods have been explored for the synthesis of 3,4-Dihydro-1H-quinoxalin-2-ones. A prevalent approach involves the reaction of o-phenylenediamine with dialkyl acetylenedicarboxylates under solvent-free conditions. [] This method proves economical and environmentally friendly. Another route utilizes the reaction of o-phenylenediamines with ethyl bromoacetate in a basic medium under mild conditions. []
Q2: Are there any reported biological activities of 3,4-Dihydro-1H-quinoxalin-2-ones?
A2: Yes, research suggests that some 3,4-Dihydro-1H-quinoxalin-2-ones and their derivatives exhibit antibacterial activity against both Gram-positive and Gram-negative bacteria. []
Q3: Can you provide an example of a specific 3,4-Dihydro-1H-quinoxalin-2-one derivative and its synthesis?
A3: Certainly. 7-Benzoyl-1H-quinoxalin-2-one (4) was synthesized by reacting 4-benzoyl-1,2-diaminobenzene with ethyl bromoacetate under basic conditions. []
Q4: What are the structural characteristics of this compound derivatives?
A4: These compounds are characterized by a quinoxaline core structure with a carbonyl group at the 2-position and a saturated six-membered ring fused to the benzene ring. Derivatives can have various substituents on the benzene ring and the nitrogen atoms. []
Q5: Are there alternative synthetic routes to specific derivatives, for instance, those with fluorine substituents?
A5: Yes, 6,8-substituted-5,7-difluoro-3,4-dihydro-1H-quinoxalin-2-ones have been synthesized via reductive cyclization of 2,4,6-substituted-3,5-difluoronitrobenzenes. [] This method allows for the incorporation of fluorine atoms into the structure.
Q6: Has sulfamic acid been used in the synthesis of this compound derivatives?
A6: Yes, sulfamic acid has been successfully employed as an effective catalyst for the synthesis of β-enaminoketone derivatives, including 3-(2-oxo-2-arylethylidene)-3,4-dihydro-1H-quinoxalin-2-ones. [] The reactions were conducted under solvent-free conditions using o-phenylenediamine and ethyl 2,4-dioxo-4-arylbutyrate as starting materials.
Q7: What analytical techniques are used to characterize 3,4-Dihydro-1H-quinoxalin-2-ones?
A7: Various spectroscopic techniques are employed to confirm the structure of these compounds. These include Infrared (IR) spectroscopy, proton Nuclear Magnetic Resonance (1H NMR) spectroscopy, and carbon-13 Nuclear Magnetic Resonance (13C NMR) spectroscopy. Additionally, mass spectrometry is often utilized to determine the molecular weight. [, ] X-ray crystallography has also been used to confirm the molecular structures of some derivatives. [, ]
Q8: Beyond antibacterial activity, are there other potential applications for 3,4-Dihydro-1H-quinoxalin-2-ones?
A8: Research suggests that Phenylethylidene-3,4-dihydro-1H-quinoxalin-2-ones show promise as building blocks for Cu2+ recognition. [] This opens avenues for their use in sensor development or metal ion extraction.
Disclaimer and Information on In-Vitro Research Products
Please be aware that all articles and product information presented on BenchChem are intended solely for informational purposes. The products available for purchase on BenchChem are specifically designed for in-vitro studies, which are conducted outside of living organisms. In-vitro studies, derived from the Latin term "in glass," involve experiments performed in controlled laboratory settings using cells or tissues. It is important to note that these products are not categorized as medicines or drugs, and they have not received approval from the FDA for the prevention, treatment, or cure of any medical condition, ailment, or disease. We must emphasize that any form of bodily introduction of these products into humans or animals is strictly prohibited by law. It is essential to adhere to these guidelines to ensure compliance with legal and ethical standards in research and experimentation.