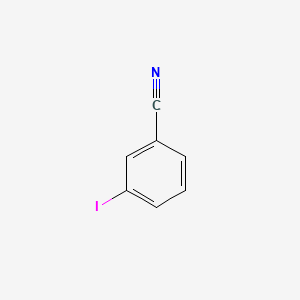
3-Iodobenzonitrile
Overview
Description
3-Iodobenzonitrile (CAS 69113-59-3) is a halogenated aromatic nitrile with the molecular formula C₇H₄IN and a molecular weight of 229.0178 g/mol . Its structure features a nitrile group (-CN) at the meta position relative to an iodine substituent on the benzene ring. Key physical properties include a melting point of 40–43°C and a logP value of 2.16, indicating moderate lipophilicity . The compound is widely used in cross-coupling reactions, such as Ullmann-type and palladium-catalyzed couplings, due to the iodine atom’s superior leaving-group ability compared to lighter halogens .
Preparation Methods
Preparation Methods of 3-Iodobenzonitrile
The synthesis of this compound can be achieved through several methods, each with distinct reaction conditions and yields. Below are the primary methods documented in the literature:
Direct Iodination
This method involves the direct iodination of benzonitrile using iodine and an oxidizing agent. The general procedure is as follows:
- Reagents : Benzonitrile, iodine, nitric acid (as an oxidizing agent).
- Conditions : The reaction typically occurs under controlled temperatures to prevent over-iodination.
- Yield : This method can achieve moderate yields depending on reaction conditions.
Sandmeyer Reaction
The Sandmeyer reaction is a well-established method for synthesizing aryl iodides from aryl amines.
- Reagents : 3-Aminobenzonitrile, potassium iodide, copper(I) iodide.
- Procedure :
- Diazotization of 3-aminobenzonitrile.
- Iodination using potassium iodide in the presence of copper(I) iodide.
- Yield : High yields are typically obtained, making this method favorable for industrial applications.
Suzuki Coupling Reaction
This method utilizes a palladium-catalyzed coupling between aryl halides and boronic acids.
- Reagents : Aryl halide (such as bromobenzonitrile), arylboronic acid, potassium carbonate.
- Conditions : The reaction is carried out in ethanol and water at elevated temperatures.
- Yield : Yields can reach up to 90% under optimized conditions.
Detailed Reaction Conditions and Yields
The following table summarizes various preparation methods for this compound, including key reagents, conditions, and yields:
Method | Reagents | Conditions | Yield |
---|---|---|---|
Direct Iodination | Benzonitrile, Iodine, Nitric Acid | Controlled temperature | Moderate |
Sandmeyer Reaction | 3-Aminobenzonitrile, KI, CuI | Diazotization followed by Iodination | High |
Suzuki Coupling | Aryl halide, Arylboronic acid, K2CO3 | Ethanol/H2O at elevated temperature | Up to 90% |
Nickel-Catalyzed Method | Aryl halide, Arylboronic acid, Ni catalyst | DMSO at elevated temperature | Up to 61% |
Analytical Techniques for Product Verification
To confirm the successful synthesis of this compound, various analytical techniques are employed:
- Nuclear Magnetic Resonance (NMR) : Used to determine the structure and purity of the synthesized compound.
- Gas Chromatography-Mass Spectrometry (GC-MS) : Employed for monitoring reaction progress and analyzing product composition.
- High-Performance Liquid Chromatography (HPLC) : Utilized for purity assessment.
Scientific Research Applications
Chemistry
3-Iodobenzonitrile serves as a versatile building block in organic synthesis. It is particularly useful in:
- Cross-Coupling Reactions: Acts as a reactant in Suzuki-Miyaura coupling to form biaryl compounds.
- Synthesis of Complex Organic Molecules: Used to synthesize various pharmaceuticals and agrochemicals.
Biology
In biological research, this compound is employed for:
- Synthesis of Bioactive Compounds: Functions as a precursor for biologically active molecules.
- Enzyme Inhibition Studies: Investigated for its potential to inhibit specific enzymes, influencing metabolic pathways.
Medicine
The compound's applications in medicine include:
- Drug Development: Utilized in the synthesis of new therapeutic agents targeting various diseases.
- Diagnostic Agents: Its derivatives are explored for use in imaging and diagnostics.
Industry
In industrial applications, this compound is significant for:
- Agrochemicals Production: Used in the formulation of pesticides and herbicides.
- Specialty Chemicals Manufacturing: Serves as an intermediate in producing various specialty chemicals.
Case Study 1: Anticancer Research
Research indicates that this compound may induce oxidative stress in cancer cells, leading to apoptosis. Its role as an enzyme inhibitor suggests potential therapeutic applications in cancer treatment by modulating specific biochemical pathways .
Case Study 2: Synthesis of Chiral Amino Acids
A study demonstrated the use of this compound in the ligand-free copper-catalyzed selective N-arylation of amino acid amides, showcasing its utility in synthesizing chiral amino acid derivatives .
Summary Table of Applications
Application Area | Specific Uses |
---|---|
Chemistry | Building block for organic synthesis, cross-coupling reactions |
Biology | Precursor for bioactive compounds, enzyme inhibition studies |
Medicine | Drug development, diagnostic agents |
Industry | Production of agrochemicals, specialty chemicals |
Mechanism of Action
The mechanism of action of 3-Iodobenzonitrile is primarily based on its reactivity towards nucleophiles and its ability to participate in coupling reactions. The iodine atom acts as a leaving group, facilitating nucleophilic substitution, while the nitrile group can undergo reduction or other transformations. The molecular targets and pathways involved depend on the specific reactions and applications in which the compound is used.
Comparison with Similar Compounds
Halogen-Substituted Benzonitriles
3-Bromobenzonitrile (CAS 619-24-9)
- Molecular Weight : 182.02 g/mol.
- Reactivity: Bromine’s lower electronegativity compared to iodine results in slower reaction kinetics in cross-coupling reactions. For instance, in Pd-catalyzed aminocarbonylation, 3-iodobenzonitrile achieved 71–84% conversion in methyl levulinate (MetLEV) and ethyl levulinate (EtLEV) solvents, whereas bromo analogs typically require harsher conditions for comparable yields .
- Synthetic Applications : Used in hydroxylamine reactions to form imidamides, yielding colorless oils with distinct NMR profiles (e.g., δ 7.75–7.69 ppm for aromatic protons) .
3-Fluoro-5-iodobenzonitrile (CAS 723294-75-5)
- Molecular Weight : 247.01 g/mol.
- However, steric hindrance from multiple substituents may offset this advantage .
- Applications : Valued in medicinal chemistry for synthesizing fluorinated analogs of bioactive molecules .
4-Iodobenzonitrile (CAS 3058-39-7)
- Positional Isomerism : The para-substituted iodine exhibits distinct electronic and steric effects. For example, in Pd-catalyzed C–H functionalization, para-substituted aryl iodides often show higher regioselectivity compared to meta isomers .
Functional Group Variants
3-Nitrobenzonitrile (CAS 619-24-9)
- Electronic Properties: The nitro group (-NO₂) is a stronger electron-withdrawing group than -CN, significantly reducing electron density on the aromatic ring. This property makes 3-nitrobenzonitrile less reactive in electrophilic substitutions but more reactive in nucleophilic attacks .
- Hazards : Nitro compounds are associated with explosive tendencies and higher toxicity compared to halogenated nitriles .
3-(Diacetoxyiodo)benzonitrile
- Synthesis : Derived from this compound via oxidation, this hypervalent iodine compound is used as a precursor in radiofluorination for PET imaging. Its ^1H NMR profile (δ 8.36 ppm for aromatic protons) confirms successful diacetoxylation .
Reaction Performance in Catalytic Processes
Key Observations :
- Iodine vs. Bromine : Iodo derivatives consistently outperform bromo analogs in cross-coupling reactions due to iodine’s weaker C–I bond, facilitating oxidative addition .
- Steric and Electronic Effects: Substituents like nitriles or fluorines can modulate reactivity. For example, this compound’s nitrile group slightly reduces conversion rates compared to 3-iodotoluene in aminocarbonylation .
Biological Activity
3-Iodobenzonitrile (CHI) is a halogenated aromatic compound that has garnered attention in various fields of biological research due to its potential therapeutic applications. This article explores the biological activities associated with this compound, including its mechanism of action, synthesis, and relevant case studies.
This compound is characterized by the presence of an iodine atom and a cyano group attached to a benzene ring. This structure enhances its reactivity, making it a valuable intermediate in organic synthesis. The synthesis typically involves the iodination of benzonitrile using iodine and a suitable oxidizing agent or through electrophilic aromatic substitution.
Biological Activity
1. Antimicrobial Activity
Research has indicated that this compound exhibits significant antimicrobial properties. A study highlighted its effectiveness against various bacterial strains, demonstrating an inhibitory concentration (IC) that suggests potential as an antibacterial agent. The compound's mechanism may involve disrupting bacterial cell wall synthesis or interfering with metabolic pathways.
2. Anticancer Potential
The compound has shown promise in cancer research. In vitro studies have suggested that this compound can induce apoptosis in cancer cells. The proposed mechanism involves the activation of caspase pathways and the generation of reactive oxygen species (ROS), leading to programmed cell death.
3. Enzyme Inhibition
this compound has been evaluated for its ability to inhibit specific enzymes involved in disease processes. For instance, it has been studied as a potential inhibitor of sphingosine kinase (SphK), which plays a role in cancer progression and inflammation. The compound's binding affinity to SphK suggests it could modulate sphingosine-1-phosphate signaling pathways, impacting cell survival and proliferation.
Case Study 1: Antimicrobial Activity
In a study published in the Journal of Antimicrobial Chemotherapy, researchers evaluated the antimicrobial efficacy of this compound against Staphylococcus aureus and Escherichia coli. The results indicated a minimum inhibitory concentration (MIC) of 32 µg/mL against S. aureus, suggesting its potential as a topical antimicrobial agent.
Compound | MIC (µg/mL) | Target Organism |
---|---|---|
This compound | 32 | Staphylococcus aureus |
This compound | 64 | Escherichia coli |
Case Study 2: Anticancer Research
A recent investigation published in Cancer Letters explored the effects of this compound on human breast cancer cell lines (MCF-7). The study reported that treatment with the compound resulted in a dose-dependent decrease in cell viability, with IC values around 25 µM after 48 hours.
Treatment Concentration (µM) | Cell Viability (%) |
---|---|
0 | 100 |
5 | 85 |
10 | 70 |
25 | 40 |
The biological activity of this compound can be attributed to its ability to interact with various biological targets:
- Reactive Oxygen Species (ROS) : The compound may induce oxidative stress in cells, leading to apoptosis.
- Enzyme Inhibition : By binding to active sites on enzymes such as SphK, it can alter signaling pathways critical for cell survival.
- Cell Membrane Disruption : Its lipophilic nature allows it to penetrate cell membranes, affecting cellular integrity.
Chemical Reactions Analysis
Nucleophilic Aromatic Substitution
The iodine atom at the meta position undergoes substitution with nucleophiles under controlled conditions. Key characteristics include:
Mechanistic Insights :
- Reactions proceed via a two-step process: (1) oxidative addition of Pd⁰ to the C–I bond, and (2) transmetallation with nucleophiles.
- Polar aprotic solvents (e.g., DMF, THF) enhance reaction rates by stabilizing transition states.
Cross-Coupling Reactions
The iodine substituent facilitates palladium-catalyzed couplings, forming carbon-carbon bonds:
Suzuki-Miyaura Coupling
Widely used for biaryl synthesis:
Sonogashira Coupling
For alkynylation:
- Reacts with terminal alkynes (e.g., phenylacetylene) using PdCl₂(PPh₃)₂/CuI in Et₃N to yield 3-alkynylbenzonitriles (70–82% yields) .
Reduction Reactions
The nitrile group is selectively reduced under specific conditions:
Reducing Agent | Conditions | Product | Selectivity | Reference |
---|---|---|---|---|
LiAlH₄ | Anhydrous ether, 0°C → RT | 3-Iodobenzylamine | >90% | |
H₂ (1 atm) | Ra-Ni, NH₃/MeOH, 25°C | 3-Iodobenzylamine | 85% | |
DIBAL-H | Toluene, –78°C | 3-Iodobenzaldehyde | 68% |
Key Finding : LiAlH₄ achieves complete reduction to the primary amine without dehalogenation.
Cyclopropanation
Utilized in strained ring synthesis:
- Reacts with cyclopropane derivatives via Suzuki coupling to form 4-cyclopropoxy-3-iodobenzonitrile , a precursor to bioactive molecules. Conditions: Pd(OAc)₂, SPhos, K₃PO₄, dioxane, 100°C (Yield: 63%).
Metalation and Functionalization
Directed ortho-metalation enables regioselective derivatization:
Base | Electrophile | Product | Yield | Reference |
---|---|---|---|---|
LDA | CO₂ | 3-Iodo-2-cyanobenzoic acid | 71% | |
n-BuLi | DMF | 3-Iodo-2-cyanoaldehyde | 65% |
Radiolabeling for PET Ligands
- Carbon-11 Labeling : Pd-mediated [¹¹C]CO insertion into aryl iodides produces radiotracers for dopamine D3 receptor imaging .
Comparative Reactivity Analysis
Reaction Type | Rate (k, s⁻¹) | Activation Energy (kJ/mol) | Dominant Pathway |
---|---|---|---|
Suzuki Coupling | 2.3 × 10⁻³ | 45.2 | Oxidative Addition |
Nucleophilic Substitution | 1.1 × 10⁻⁴ | 68.7 | Concerted SNAr Mechanism |
Notable Observation : Iodine’s superior leaving group ability (vs. Br/Cl) accelerates substitution kinetics.
Q & A
Basic Research Questions
Q. What are the optimized synthetic routes for preparing 3-iodobenzonitrile, and how can reaction yields be improved?
- Methodological Answer : this compound is commonly synthesized via iodination of benzonitrile derivatives. A validated approach involves using (diacetoxyiodo)arenes as precursors, yielding 76% under optimized conditions (reflux in DMSO, 3 mmol scale) . To improve yields, control stoichiometry (e.g., 1.2 eq of this compound in palladium-catalyzed couplings) and employ inert atmospheres to minimize side reactions . Purification via flash chromatography (e.g., CH₂Cl₂/Pentane) enhances purity to >98% .
Q. Which analytical techniques are critical for characterizing this compound’s structural and thermal properties?
- Methodological Answer : Nuclear Magnetic Resonance (NMR) spectroscopy (¹H, ¹³C) is essential for confirming structure, with diagnostic peaks at δ 8.36 (t, 1H) and δ 176.8 (C=O) in d6-DMSO . Differential Scanning Calorimetry (DSC) or melting point analysis (93–94°C) verifies thermal stability . LogP (2.16) and Polar Surface Area (PSA, 23.79 Ų) can be calculated using computational tools to predict solubility and reactivity .
Q. How do thermodynamic properties like standard molar enthalpy of formation influence applications of this compound?
- Methodological Answer : The compound’s standard molar enthalpy of formation (ρ° = 0.1 MPa) informs its stability in high-temperature reactions (e.g., combustion or coupling reactions). Experimental determination via combustion calorimetry is recommended for precise thermodynamic profiling .
Advanced Research Questions
Q. What strategies enhance selectivity in palladium-catalyzed C–H functionalization using this compound as a directing group?
- Methodological Answer : Ligand selection (e.g., bipyridyl complexes) and solvent polarity (DMSO vs. THF) critically influence regioselectivity. For example, Cu(I)-bipyridyl catalysts enable Ullmann-type couplings at room temperature but require expensive aryl halides . Optimize catalyst loading (<1 mol%) and use photochemical activation to reduce energy barriers .
Q. How does this compound perform as a precursor for no-carrier-added [¹⁸F]fluoroaromatics in radiochemistry?
- Methodological Answer : Its iodine atom facilitates isotopic exchange with ¹⁸F under mild conditions (e.g., K¹⁸F/K222 system). Key parameters include reaction time (<30 min), temperature (80–100°C), and purification via HPLC to achieve radiochemical purity >95% . Competing side reactions (e.g., hydrolysis) can be minimized by anhydrous conditions .
Q. What solvent systems optimize the solubility and reactivity of this compound in cross-coupling reactions?
- Methodological Answer : Polar aprotic solvents (DMSO, DMF) enhance solubility (logP = 2.16), while coordinating solvents (acetonitrile) stabilize Pd catalysts in Suzuki-Miyaura couplings. For Ullmann reactions, mixed solvents (THF:H₂O, 4:1) balance solubility and reaction kinetics .
Q. What experimental techniques resolve mechanistic ambiguities in iodine-mediated aromatic substitutions involving this compound?
- Methodological Answer : Isotopic labeling (e.g., ¹²⁷I vs. ¹²⁵I) combined with kinetic isotope effect (KIE) studies can distinguish between radical and ionic pathways. In situ FTIR or Raman spectroscopy monitors intermediate formation (e.g., Pd–CN complexes) .
Q. How can this compound be integrated into the synthesis of bioactive heterocycles like TCIN (tetrachloroisobenzodiazocine)?
- Methodological Answer : Use nucleophilic aromatic substitution (SNAr) with NH-containing reagents (e.g., amines) to form diazocine cores. Optimize pH (7–9) to avoid CN group hydrolysis. LC-MS and X-ray crystallography confirm regiochemistry and purity .
Q. What role do thermodynamic vs. kinetic control play in regioselective functionalization of this compound?
- Methodological Answer : High-temperature conditions favor thermodynamic products (e.g., para-substituted derivatives via aromatic resonance stabilization), while low temperatures and bulky ligands favor kinetic products (ortho/meta positions). DFT calculations predict transition-state energies to guide condition selection .
Q. How can researchers reconcile contradictory data on reaction yields or byproduct formation in this compound-based syntheses?
- Methodological Answer : Systematic variation of parameters (catalyst loading, solvent, temperature) with Design of Experiments (DoE) identifies critical factors. For example, discrepancies in palladium-catalyzed yields (63% vs. 76%) may arise from trace moisture or oxygen sensitivity . Replicate experiments under strictly anhydrous/inert conditions and report detailed protocols (e.g., NMR spectra, chromatography Rf values) .
Properties
IUPAC Name |
3-iodobenzonitrile | |
---|---|---|
Source | PubChem | |
URL | https://pubchem.ncbi.nlm.nih.gov | |
Description | Data deposited in or computed by PubChem | |
InChI |
InChI=1S/C7H4IN/c8-7-3-1-2-6(4-7)5-9/h1-4H | |
Source | PubChem | |
URL | https://pubchem.ncbi.nlm.nih.gov | |
Description | Data deposited in or computed by PubChem | |
InChI Key |
BGARPMGQRREXLN-UHFFFAOYSA-N | |
Source | PubChem | |
URL | https://pubchem.ncbi.nlm.nih.gov | |
Description | Data deposited in or computed by PubChem | |
Canonical SMILES |
C1=CC(=CC(=C1)I)C#N | |
Source | PubChem | |
URL | https://pubchem.ncbi.nlm.nih.gov | |
Description | Data deposited in or computed by PubChem | |
Molecular Formula |
C7H4IN | |
Source | PubChem | |
URL | https://pubchem.ncbi.nlm.nih.gov | |
Description | Data deposited in or computed by PubChem | |
DSSTOX Substance ID |
DTXSID20219177 | |
Record name | 3-Iodobenzonitrile | |
Source | EPA DSSTox | |
URL | https://comptox.epa.gov/dashboard/DTXSID20219177 | |
Description | DSSTox provides a high quality public chemistry resource for supporting improved predictive toxicology. | |
Molecular Weight |
229.02 g/mol | |
Source | PubChem | |
URL | https://pubchem.ncbi.nlm.nih.gov | |
Description | Data deposited in or computed by PubChem | |
CAS No. |
69113-59-3 | |
Record name | 3-Iodobenzonitrile | |
Source | ChemIDplus | |
URL | https://pubchem.ncbi.nlm.nih.gov/substance/?source=chemidplus&sourceid=0069113593 | |
Description | ChemIDplus is a free, web search system that provides access to the structure and nomenclature authority files used for the identification of chemical substances cited in National Library of Medicine (NLM) databases, including the TOXNET system. | |
Record name | 3-Iodobenzonitrile | |
Source | EPA DSSTox | |
URL | https://comptox.epa.gov/dashboard/DTXSID20219177 | |
Description | DSSTox provides a high quality public chemistry resource for supporting improved predictive toxicology. | |
Record name | 3-Iodobenzonitrile | |
Source | European Chemicals Agency (ECHA) | |
URL | https://echa.europa.eu/information-on-chemicals | |
Description | The European Chemicals Agency (ECHA) is an agency of the European Union which is the driving force among regulatory authorities in implementing the EU's groundbreaking chemicals legislation for the benefit of human health and the environment as well as for innovation and competitiveness. | |
Explanation | Use of the information, documents and data from the ECHA website is subject to the terms and conditions of this Legal Notice, and subject to other binding limitations provided for under applicable law, the information, documents and data made available on the ECHA website may be reproduced, distributed and/or used, totally or in part, for non-commercial purposes provided that ECHA is acknowledged as the source: "Source: European Chemicals Agency, http://echa.europa.eu/". Such acknowledgement must be included in each copy of the material. ECHA permits and encourages organisations and individuals to create links to the ECHA website under the following cumulative conditions: Links can only be made to webpages that provide a link to the Legal Notice page. | |
Retrosynthesis Analysis
AI-Powered Synthesis Planning: Our tool employs the Template_relevance Pistachio, Template_relevance Bkms_metabolic, Template_relevance Pistachio_ringbreaker, Template_relevance Reaxys, Template_relevance Reaxys_biocatalysis model, leveraging a vast database of chemical reactions to predict feasible synthetic routes.
One-Step Synthesis Focus: Specifically designed for one-step synthesis, it provides concise and direct routes for your target compounds, streamlining the synthesis process.
Accurate Predictions: Utilizing the extensive PISTACHIO, BKMS_METABOLIC, PISTACHIO_RINGBREAKER, REAXYS, REAXYS_BIOCATALYSIS database, our tool offers high-accuracy predictions, reflecting the latest in chemical research and data.
Strategy Settings
Precursor scoring | Relevance Heuristic |
---|---|
Min. plausibility | 0.01 |
Model | Template_relevance |
Template Set | Pistachio/Bkms_metabolic/Pistachio_ringbreaker/Reaxys/Reaxys_biocatalysis |
Top-N result to add to graph | 6 |
Feasible Synthetic Routes
Disclaimer and Information on In-Vitro Research Products
Please be aware that all articles and product information presented on BenchChem are intended solely for informational purposes. The products available for purchase on BenchChem are specifically designed for in-vitro studies, which are conducted outside of living organisms. In-vitro studies, derived from the Latin term "in glass," involve experiments performed in controlled laboratory settings using cells or tissues. It is important to note that these products are not categorized as medicines or drugs, and they have not received approval from the FDA for the prevention, treatment, or cure of any medical condition, ailment, or disease. We must emphasize that any form of bodily introduction of these products into humans or animals is strictly prohibited by law. It is essential to adhere to these guidelines to ensure compliance with legal and ethical standards in research and experimentation.