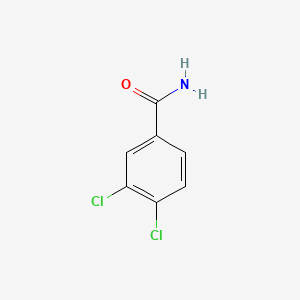
3,4-Dichlorobenzamide
Overview
Description
3,4-Dichlorobenzamide is an organic compound with the molecular formula C7H5Cl2NO and a molecular weight of 190.027 g/mol . It is a derivative of benzamide, where two chlorine atoms are substituted at the 3rd and 4th positions of the benzene ring. This compound is known for its applications in various fields, including chemistry, biology, and industry.
Preparation Methods
Synthetic Routes and Reaction Conditions: 3,4-Dichlorobenzamide can be synthesized through the reaction of 3,4-dichlorobenzoic acid with oxalyl chloride in the presence of N,N-dimethylformamide (DMF) at 0°C to 25°C for 12 hours. The resulting product is then treated with ammonium hydroxide in dichloromethane at 0°C for 1 hour to yield this compound .
Industrial Production Methods: In industrial settings, the synthesis of this compound follows similar routes but on a larger scale. The reaction conditions are optimized to ensure high yield and purity of the product. The use of continuous flow reactors and automated systems helps in scaling up the production while maintaining consistency and quality.
Chemical Reactions Analysis
Types of Reactions: 3,4-Dichlorobenzamide undergoes various chemical reactions, including:
Substitution Reactions: The chlorine atoms can be substituted with other nucleophiles under appropriate conditions.
Reduction Reactions: The amide group can be reduced to an amine using reducing agents like lithium aluminum hydride.
Oxidation Reactions: The compound can be oxidized to form corresponding carboxylic acids or other oxidized derivatives.
Common Reagents and Conditions:
Substitution: Reagents like sodium hydroxide or potassium hydroxide in aqueous or alcoholic solutions.
Reduction: Lithium aluminum hydride in anhydrous ether.
Oxidation: Potassium permanganate or chromium trioxide in acidic or basic media.
Major Products:
Substitution: Products depend on the nucleophile used, such as 3,4-dichloroaniline when ammonia is used.
Reduction: 3,4-dichloroaniline.
Oxidation: 3,4-dichlorobenzoic acid.
Scientific Research Applications
3,4-Dichlorobenzamide has a wide range of applications in scientific research:
Chemistry: Used as an intermediate in the synthesis of various organic compounds, including pharmaceuticals and agrochemicals.
Biology: Studied for its potential biological activities, including antimicrobial and herbicidal properties.
Medicine: Investigated for its potential therapeutic effects and as a building block for drug development.
Industry: Utilized in the production of dyes, pigments, and other industrial chemicals.
Mechanism of Action
The mechanism of action of 3,4-dichlorobenzamide involves its interaction with specific molecular targets and pathways. It is known to inhibit certain enzymes and disrupt cellular processes. For example, its herbicidal properties are attributed to its ability to interfere with the synthesis of essential biomolecules in plants, leading to growth inhibition and cell death .
Comparison with Similar Compounds
- 2,4-Dichlorobenzamide
- 3,5-Dichlorobenzamide
- 2,6-Dichlorobenzamide
Comparison: 3,4-Dichlorobenzamide is unique due to the specific positioning of chlorine atoms on the benzene ring, which influences its chemical reactivity and biological activity. Compared to its isomers, this compound exhibits distinct properties and applications, making it valuable in specific research and industrial contexts .
Properties
IUPAC Name |
3,4-dichlorobenzamide | |
---|---|---|
Source | PubChem | |
URL | https://pubchem.ncbi.nlm.nih.gov | |
Description | Data deposited in or computed by PubChem | |
InChI |
InChI=1S/C7H5Cl2NO/c8-5-2-1-4(7(10)11)3-6(5)9/h1-3H,(H2,10,11) | |
Source | PubChem | |
URL | https://pubchem.ncbi.nlm.nih.gov | |
Description | Data deposited in or computed by PubChem | |
InChI Key |
XURBWYCGQQXTHJ-UHFFFAOYSA-N | |
Source | PubChem | |
URL | https://pubchem.ncbi.nlm.nih.gov | |
Description | Data deposited in or computed by PubChem | |
Canonical SMILES |
C1=CC(=C(C=C1C(=O)N)Cl)Cl | |
Source | PubChem | |
URL | https://pubchem.ncbi.nlm.nih.gov | |
Description | Data deposited in or computed by PubChem | |
Molecular Formula |
C7H5Cl2NO | |
Source | PubChem | |
URL | https://pubchem.ncbi.nlm.nih.gov | |
Description | Data deposited in or computed by PubChem | |
DSSTOX Substance ID |
DTXSID00181207 | |
Record name | 3,4-Dichlorobenzamide | |
Source | EPA DSSTox | |
URL | https://comptox.epa.gov/dashboard/DTXSID00181207 | |
Description | DSSTox provides a high quality public chemistry resource for supporting improved predictive toxicology. | |
Molecular Weight |
190.02 g/mol | |
Source | PubChem | |
URL | https://pubchem.ncbi.nlm.nih.gov | |
Description | Data deposited in or computed by PubChem | |
CAS No. |
2670-38-4 | |
Record name | 3,4-Dichlorobenzamide | |
Source | ChemIDplus | |
URL | https://pubchem.ncbi.nlm.nih.gov/substance/?source=chemidplus&sourceid=0002670384 | |
Description | ChemIDplus is a free, web search system that provides access to the structure and nomenclature authority files used for the identification of chemical substances cited in National Library of Medicine (NLM) databases, including the TOXNET system. | |
Record name | 2670-38-4 | |
Source | DTP/NCI | |
URL | https://dtp.cancer.gov/dtpstandard/servlet/dwindex?searchtype=NSC&outputformat=html&searchlist=23779 | |
Description | The NCI Development Therapeutics Program (DTP) provides services and resources to the academic and private-sector research communities worldwide to facilitate the discovery and development of new cancer therapeutic agents. | |
Explanation | Unless otherwise indicated, all text within NCI products is free of copyright and may be reused without our permission. Credit the National Cancer Institute as the source. | |
Record name | 3,4-Dichlorobenzamide | |
Source | EPA DSSTox | |
URL | https://comptox.epa.gov/dashboard/DTXSID00181207 | |
Description | DSSTox provides a high quality public chemistry resource for supporting improved predictive toxicology. | |
Retrosynthesis Analysis
AI-Powered Synthesis Planning: Our tool employs the Template_relevance Pistachio, Template_relevance Bkms_metabolic, Template_relevance Pistachio_ringbreaker, Template_relevance Reaxys, Template_relevance Reaxys_biocatalysis model, leveraging a vast database of chemical reactions to predict feasible synthetic routes.
One-Step Synthesis Focus: Specifically designed for one-step synthesis, it provides concise and direct routes for your target compounds, streamlining the synthesis process.
Accurate Predictions: Utilizing the extensive PISTACHIO, BKMS_METABOLIC, PISTACHIO_RINGBREAKER, REAXYS, REAXYS_BIOCATALYSIS database, our tool offers high-accuracy predictions, reflecting the latest in chemical research and data.
Strategy Settings
Precursor scoring | Relevance Heuristic |
---|---|
Min. plausibility | 0.01 |
Model | Template_relevance |
Template Set | Pistachio/Bkms_metabolic/Pistachio_ringbreaker/Reaxys/Reaxys_biocatalysis |
Top-N result to add to graph | 6 |
Feasible Synthetic Routes
A: Research suggests that certain asymmetric N,N-dialkyl-3,4-dichlorobenzamides exhibit phytotoxic activity, potentially acting as herbicides. Specifically, compounds with a sec-butyl, 1-methylbutyl, or 1-ethylpropyl group, along with a strategically chosen second substituent on the N-amide, showed selective phytotoxicity towards weed species like Echinochloa and Setaria. [] Further research is needed to optimize these compounds for efficacy and safety in agricultural settings.
A: Studies exploring the structure-activity relationship (SAR) of N-(allylcarbamothioyl)-3-chlorobenzamide (BATU-02) and N-(allylcarbamothioyl)-3,4-dichlorobenzamide (BATU-04) revealed promising results against human breast cancer cell line (T47D). [] While the exact mechanism is still under investigation, molecular docking simulations suggest that these compounds might interact with the Epidermal Growth Factor Receptor (EGFR), a key protein involved in cancer cell growth and proliferation. The presence of chlorine atoms and the specific thiourea moiety in BATU-02 and BATU-04 appear crucial for their enhanced activity compared to the reference drug 5-fluorouracil. []
A: Research indicates that incorporating thianthrene units, synthesized from 3,4-Dichlorobenzamide, into polyamides can enhance their solubility compared to analogous poly(thioether amide)s. [] These thianthrene-based polyamides exhibited good thermal stability, making them potentially suitable for applications requiring durability at elevated temperatures. []
A: While promising, the research on this compound derivatives is still in its early stages. One challenge is the potential for antimicrobial resistance. [] Developing strategies to mitigate resistance development will be crucial for long-term therapeutic success. Additionally, thorough toxicological studies are necessary to understand the safety profile and potential long-term effects of these compounds. []
Disclaimer and Information on In-Vitro Research Products
Please be aware that all articles and product information presented on BenchChem are intended solely for informational purposes. The products available for purchase on BenchChem are specifically designed for in-vitro studies, which are conducted outside of living organisms. In-vitro studies, derived from the Latin term "in glass," involve experiments performed in controlled laboratory settings using cells or tissues. It is important to note that these products are not categorized as medicines or drugs, and they have not received approval from the FDA for the prevention, treatment, or cure of any medical condition, ailment, or disease. We must emphasize that any form of bodily introduction of these products into humans or animals is strictly prohibited by law. It is essential to adhere to these guidelines to ensure compliance with legal and ethical standards in research and experimentation.