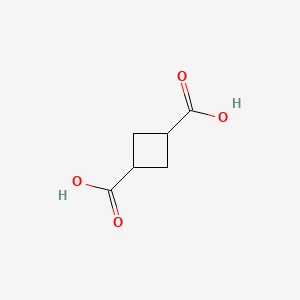
cyclobutane-1,3-dicarboxylic acid
Overview
Description
Cyclobutane-1,3-dicarboxylic acid (CBDA, C₆H₈O₄) is a semi-rigid alicyclic compound featuring two carboxylic acid groups at the 1,3-positions of a cyclobutane ring. Its structural rigidity and chemical stability make it a valuable building block in materials science and organic synthesis.
Preparation Methods
Photodimerization of Trans-Cinnamic Acid
One of the most common and efficient methods for synthesizing cyclobutane-1,3-dicarboxylic acid involves the photodimerization of trans-cinnamic acid. This method utilizes UV light to induce a dimerization reaction that forms the desired cyclobutane structure.
Procedure : Trans-cinnamic acid is exposed to UV light, which causes it to undergo a [2 + 2] cycloaddition reaction, resulting in the formation of this compound.
Advantages : This method is favored due to its simplicity and the availability of starting materials. The reaction typically occurs in solid-state conditions, enhancing stability and yield.
Yield : The photodimerization process has been reported to yield high purity products with minimal byproducts.
Knoevenagel Condensation
Another synthetic route involves the Knoevenagel condensation reaction between furfural and malonic acid.
Procedure : In this method, furfural reacts with malonic acid in the presence of a base catalyst to form an intermediate compound. Subsequent decarboxylation leads to the formation of this compound.
Advantages : This method allows for the use of readily available reagents and can be performed under mild conditions.
Yield : While specific yield data can vary based on reaction conditions, this method generally provides moderate yields.
Industrial Production Methods
In industrial settings, scalable synthetic routes are developed to ensure high yield and purity of this compound.
Common Techniques : The industrial synthesis often employs variations of the photodimerization method or adaptations of the Knoevenagel condensation to optimize production efficiency.
Yield and Purity : These methods are designed for large-scale production while maintaining high standards for purity and consistency in the final product.
Other Notable Methods
Additional methods have been explored in research settings:
Cycloaddition Reactions : Research has indicated that cycloaddition reactions involving various unsaturated compounds can also lead to the formation of cyclobutane derivatives.
Biomass-Derived Starting Materials : Recent advancements have focused on synthesizing cyclobutane-containing diacid building blocks from biomass-derived materials using clean photocycloaddition processes.
The following table summarizes key aspects of the various preparation methods for this compound:
Method | Key Steps | Advantages | Typical Yield |
---|---|---|---|
Photodimerization | UV-induced dimerization of trans-cinnamic acid | High purity, simple procedure | High |
Knoevenagel Condensation | Reaction between furfural and malonic acid | Mild conditions, readily available reagents | Moderate |
Industrial Production | Scalable adaptations of photodimerization or Knoevenagel | High yield and purity | Varies |
Biomass-Derived Synthesis | Clean photocycloaddition from biomass | Sustainable materials | Varies |
Chemical Reactions Analysis
Types of Reactions:
Oxidation: cyclobutane-1,3-dicarboxylic acid can undergo oxidation reactions, typically using strong oxidizing agents like potassium permanganate or chromium trioxide.
Reduction: Reduction reactions can be carried out using reducing agents such as lithium aluminum hydride or hydrogen gas in the presence of a catalyst.
Substitution: The compound can participate in substitution reactions, where one of the carboxyl groups can be replaced by other functional groups under appropriate conditions.
Common Reagents and Conditions:
Oxidation: Potassium permanganate (KMnO₄), chromium trioxide (CrO₃)
Reduction: Lithium aluminum hydride (LiAlH₄), hydrogen gas (H₂) with a catalyst
Substitution: Various nucleophiles and electrophiles depending on the desired substitution
Major Products:
Oxidation: Formation of diketones or other oxidized derivatives
Reduction: Formation of alcohols or alkanes
Substitution: Formation of substituted cyclobutane derivatives
Scientific Research Applications
Polymer Synthesis
CBDA serves as a semi-rigid building block in the development of polymers, particularly polyesters. Research has demonstrated that CBDA can be polymerized with various diols to produce a series of cyclobutane-containing polyesters. For example, poly(ethylene-α-truxillate) and poly(1,4-butylene-α-truxillate) are synthesized through condensation reactions involving CBDA. These polymers exhibit thermal and chemical stabilities comparable to polyethylene terephthalate (PET), which is widely used in plastic production .
Table 1: Properties of Cyclobutane-Containing Polyesters
Polymer Type | Thermal Stability | Chemical Stability | Application Areas |
---|---|---|---|
Poly(ethylene-α-truxillate) | High | High | Packaging, textiles |
Poly(1,4-butylene-α-truxillate) | Moderate | Moderate | Automotive parts, coatings |
Photochemical Applications
CBDA has shown remarkable stability under UV irradiation, making it suitable for photochemical applications. Studies indicate that when exposed to sunlight or UV light, CBDA does not undergo significant degradation or structural changes. This property is beneficial for applications requiring long-lasting materials that can withstand environmental stressors .
Thermally Recyclable Materials
Research into the thermal properties of CBDA suggests that it can be utilized in the development of thermally recyclable or degradable materials. The cyclobutane ring can be cleaved upon heating, allowing for the recycling of materials synthesized from CBDA. This feature aligns with the growing demand for sustainable materials in various industries .
Metal-Organic Frameworks (MOFs)
CBDA also finds application in the synthesis of metal-organic frameworks (MOFs). Its ability to form stable coordination bonds with metal ions makes it an ideal candidate for creating porous structures that can be used in gas storage and separation technologies. The unique geometry of the cyclobutane ring contributes to the structural integrity and functionality of these frameworks .
Coordination Polymers
The reactivity of CBDA's carboxylic acid groups allows it to serve as a ligand in coordination chemistry. This property enables the formation of coordination polymers with various metal ions, which can have applications in catalysis and material science .
Case Study 1: Development of Polyesters
A recent study synthesized five different polyesters using CBDA as a monomer. The resulting materials were characterized using techniques such as NMR and FT-IR spectroscopy, revealing their potential for high-performance applications in packaging and textiles due to their favorable thermal and mechanical properties .
Case Study 2: Photostability Investigation
Another investigation focused on the photostability of α-truxillic acid derived from CBDA. The study demonstrated that this compound maintained its structural integrity after prolonged exposure to UV light, suggesting its utility in outdoor applications where UV resistance is critical .
Mechanism of Action
The mechanism of action of cis-cyclobutane-1,3-dicarboxylic acid involves its interaction with specific molecular targets and pathways. The compound’s unique structure allows it to participate in various chemical reactions, influencing biological processes and material properties. For example, its ability to undergo photodimerization and other cycloaddition reactions makes it a versatile building block in synthetic chemistry .
Comparison with Similar Compounds
Comparison with Similar Cyclobutane Derivatives
Structural Isomers and Positional Derivatives
Cyclobutane-1,1-Dicarboxylic Acid
- Structure : Carboxylic groups at 1,1-positions.
- Synthesis : Prepared via cyclization of 1,3-dibromopropane with malonic acid diethyl ester, followed by hydrolysis .
- Applications: Used in platinum drug synthesis (e.g., 3-(dodecanoyloxy)cyclobutane-1,1-dicarboxylic acid) and as a ligand in vanadium(IV)-lanthanide complexes .
- Comparison : The 1,1-substitution reduces steric strain but limits conformational flexibility compared to CBDA, making it more suitable for coordination chemistry .
Cyclobutane-1,2-Dicarboxylic Acid
- Structure : Carboxylic groups at adjacent positions (1,2).
- Properties : Higher ring strain due to eclipsed substituents, leading to lower thermal stability than CBDA .
- Applications: Limited evidence, but structural analogs like bicyclo[1.1.1]pentane-1,3-dicarboxylic acid (similarity score 0.95 to CBDA) are explored in drug design for their rigidity .
cis-Cyclobutane-1,3-Dicarboxylic Acid
- Structure : Cis isomer of CBDA (CAS 2398-16-5).
- Properties : Lower symmetry and higher solubility in polar solvents compared to the trans isomer (α-truxillic acid) .
Substituted Derivatives
Acyloxy Derivatives
- Examples: 3-(dodecanoyloxy)cyclobutane-1,1-dicarboxylic acid (3c) and 3-(pentanoyloxy)cyclobutane-1,1-dicarboxylic acid (3d) .
- Synthesis : Derived via esterification with acyl chlorides.
- Applications : Used to improve drug-loading capacity in platinum-based antitumor agents .
Aryl-Substituted Derivatives
- Examples : 2,4-Di(thiophen-2-yl)cyclobutane-1,3-dicarboxylic acid (L2) and 3,4-di(thiophen-2-yl)cyclobutane-1,2-dicarboxylic acid (L4) .
- Applications : Serve as ligands in photomechanical coordination polymers for optical driving systems .
Higher Carboxylic Acid Derivatives
Cyclobutane-1,1,3,3-Tetracarboxylic Acid
- Structure : Four carboxylic groups (C₈H₈O₈).
- Properties : Increased acidity and coordination sites compared to CBDA, making it suitable for MOFs and supramolecular assemblies .
Non-Cyclobutane Analogues
Cyclopentane and Cyclohexane Derivatives
- Examples: 1-Aminocyclohexane-trans-1,3-dicarboxylic acid: Modulates glutamate uptake in pineal gland microvesicles . 1-Aminocyclopentane-1,3-dicarboxylic acid (74): Acts as a metabotropic glutamate receptor agonist, reducing kynurenic acid (KYNA) levels in rat brains .
- Comparison : Larger rings (cyclopentane/cyclohexane) reduce angle strain but increase conformational flexibility, altering biological activity compared to CBDA .
Data Tables
Table 1: Structural and Functional Comparison
Biological Activity
Cyclobutane-1,3-dicarboxylic acid (CBDA) has emerged as a significant compound in materials synthesis and biological applications due to its unique structural properties and stability. This article explores the biological activity of CBDA, focusing on its synthesis, stability, potential applications, and relevant case studies.
Structural Characteristics
CBDA is characterized by its cyclobutane ring and two carboxylic acid functional groups. The compound can be synthesized through the photodimerization of trans-cinnamic acid, yielding high purity and stability under various environmental conditions. The structure allows for the formation of polymers, such as poly-α-truxillates, which exhibit semi-crystalline properties and comparable stability to well-known materials like PET (polyethylene terephthalate) .
Property | Value |
---|---|
Empirical Formula | CHO |
Molar Mass | 144.13 g/mol |
Melting Point | 155-159 °C |
Stability | High (thermal and photochemical) |
Synthesis Methods
The synthesis of CBDA primarily involves the following methods:
- Photodimerization : Utilizing UV light to promote the reaction of trans-cinnamic acid to form CBDA. This method is efficient, yielding high purity in a short time without the need for solvents .
- Continuous Flow Chemistry : Recent advancements have introduced flow chemistry techniques to scale up the production of CBDA derivatives, enhancing efficiency and reducing waste .
Stability and Reactivity
CBDA exhibits remarkable stability against thermal degradation and photochemical reactions. Studies indicate that it remains unchanged under prolonged exposure to sunlight and heating, making it suitable for various applications in materials science . Its chemical stability also allows it to withstand acidic and basic conditions without significant degradation.
Polymerization Potential
The two carboxylic acid groups in CBDA can react with diols to form a variety of polymers. These polymers have been analyzed using techniques such as NMR (Nuclear Magnetic Resonance), FT-IR (Fourier Transform Infrared Spectroscopy), and HRMS (High-Resolution Mass Spectrometry). The resulting poly-α-truxillates are semi-crystalline with potential applications in biodegradable plastics due to their environmental stability .
Case Studies
- Material Science Applications : Research has demonstrated that polymers derived from CBDA exhibit mechanical properties comparable to traditional plastics while being more environmentally friendly. For instance, poly-α-truxillates synthesized from CBDA showed promising results in tensile strength tests, indicating their potential use in packaging materials .
- Pharmaceutical Potential : Preliminary studies suggest that derivatives of CBDA may possess biological activity relevant to medicinal chemistry. The structural features of CBDA allow for modifications that could enhance bioactivity against specific targets in drug development .
Q & A
Basic Research Questions
Q. What are the established synthetic routes for cyclobutane-1,3-dicarboxylic acid (CBDA) in laboratory settings?
CBDA is commonly synthesized via [2+2] photocyclization of α-truxillic acid derivatives (e.g., trans-2,4-diphenylthis compound), leveraging ultraviolet light to induce cyclobutane ring formation . Alternative methods include base-mediated cyclization of dibromopropane derivatives with malonic acid esters, followed by hydrolysis to yield CBDA analogs . Key experimental considerations include reaction temperature (ambient to 0°C for photocyclization) and solvent selection (e.g., methanol or THF for ester hydrolysis).
Q. How is CBDA characterized structurally, particularly regarding stereochemical properties?
X-ray crystallography and NMR spectroscopy are primary tools for structural elucidation. CBDA derivatives often exhibit symmetry elements such as a center of symmetry in the cyclobutane ring, as seen in 2,4-dimethyl-CBDA, which simplifies stereochemical analysis . For enantiomeric resolution, chiral HPLC or polarimetry can be employed.
Q. What stability and handling protocols are critical for CBDA in experimental workflows?
CBDA is stable under normal laboratory conditions but degrades upon prolonged exposure to strong oxidizers or extreme pH. Storage should be in ventilated, cool environments (<25°C) away from incompatible materials (e.g., peroxides) . Personal protective equipment (PPE), including N95 masks, nitrile gloves, and eye protection, is mandatory during handling due to limited acute toxicity data .
Advanced Research Questions
Q. How can researchers overcome stereochemical challenges in synthesizing CBDA derivatives with multiple stereocenters?
Crystal engineering strategies, such as topochemical [2+2] photocycloaddition under visible light or sunlight, enable stereospecific synthesis of CBDA derivatives (e.g., rel-(1R,2R,3S,4S)-2,4-bis(3,4-dihydroxyphenyl) CBDA). Hirshfeld surface analysis and 2D intermolecular interaction fingerprints guide route design to ensure stereochemical fidelity . Solvent-free conditions and LED light sources enhance reaction efficiency and purity .
Q. What methodological approaches resolve conflicting data in CBDA reactivity studies, such as divergent oxidation or polymerization outcomes?
Contradictions in reactivity (e.g., variable photodegradation rates) can be addressed by:
- Comparative kinetic studies using UV-Vis spectroscopy under controlled wavelength exposure .
- Computational modeling (DFT) to predict reaction pathways and intermediates.
- Systematic variation of substituents (e.g., electron-withdrawing groups on phenyl rings) to isolate electronic effects .
Q. How does CBDA’s structural rigidity enhance its utility in advanced materials like metal-organic frameworks (MOFs) or degradable polymers?
CBDA’s semi-rigid cyclobutane core provides geometric constraint, enabling precise control over pore size in MOFs when coordinated with metal nodes (e.g., Zn²⁺ or Cu²⁺) . In polymers, CBDA derivatives exhibit photodegradability under specific wavelengths (e.g., 365 nm UV), making them candidates for environmentally responsive materials . Comparative studies with cyclopropane or cyclohexane analogs highlight CBDA’s superior thermal stability and mechanical strength .
Q. Data Contradiction Analysis
Q. How should researchers interpret discrepancies in reported solubility or reactivity of CBDA across studies?
Discrepancies often arise from:
- Crystallinity differences : Amorphous vs. crystalline CBDA exhibits varying solubility in DMSO or water .
- pH-dependent behavior : Carboxylic acid deprotonation at pH >5 alters solubility and reactivity .
- Isomeric impurities : Contamination with 1,1- or 1,2-dicarboxylic acid isomers can skew results . Validate purity via HPLC and elemental analysis before experimental replication.
Q. Methodological Best Practices
Q. What analytical techniques are recommended for quantifying CBDA in complex matrices (e.g., biological or polymer blends)?
- LC-MS/MS : Provides high sensitivity (detection limit ~0.1 ng/mL) using negative ion mode for carboxylic acid groups.
- FT-IR spectroscopy : Characteristic C=O stretching (1700–1750 cm⁻¹) and cyclobutane ring vibrations (800–1000 cm⁻¹) .
- Thermogravimetric analysis (TGA) : Assesses thermal stability in polymeric composites, with decomposition onset temperatures typically >200°C .
Properties
IUPAC Name |
cyclobutane-1,3-dicarboxylic acid | |
---|---|---|
Source | PubChem | |
URL | https://pubchem.ncbi.nlm.nih.gov | |
Description | Data deposited in or computed by PubChem | |
InChI |
InChI=1S/C6H8O4/c7-5(8)3-1-4(2-3)6(9)10/h3-4H,1-2H2,(H,7,8)(H,9,10) | |
Source | PubChem | |
URL | https://pubchem.ncbi.nlm.nih.gov | |
Description | Data deposited in or computed by PubChem | |
InChI Key |
WYHYNUWZLKTEEY-UHFFFAOYSA-N | |
Source | PubChem | |
URL | https://pubchem.ncbi.nlm.nih.gov | |
Description | Data deposited in or computed by PubChem | |
Canonical SMILES |
C1C(CC1C(=O)O)C(=O)O | |
Source | PubChem | |
URL | https://pubchem.ncbi.nlm.nih.gov | |
Description | Data deposited in or computed by PubChem | |
Molecular Formula |
C6H8O4 | |
Source | PubChem | |
URL | https://pubchem.ncbi.nlm.nih.gov | |
Description | Data deposited in or computed by PubChem | |
DSSTOX Substance ID |
DTXSID90178702 | |
Record name | cis-Cyclobutane-1,3-dicarboxylic acid | |
Source | EPA DSSTox | |
URL | https://comptox.epa.gov/dashboard/DTXSID90178702 | |
Description | DSSTox provides a high quality public chemistry resource for supporting improved predictive toxicology. | |
Molecular Weight |
144.12 g/mol | |
Source | PubChem | |
URL | https://pubchem.ncbi.nlm.nih.gov | |
Description | Data deposited in or computed by PubChem | |
CAS No. |
2398-16-5 | |
Record name | cis-Cyclobutane-1,3-dicarboxylic acid | |
Source | ChemIDplus | |
URL | https://pubchem.ncbi.nlm.nih.gov/substance/?source=chemidplus&sourceid=0002398165 | |
Description | ChemIDplus is a free, web search system that provides access to the structure and nomenclature authority files used for the identification of chemical substances cited in National Library of Medicine (NLM) databases, including the TOXNET system. | |
Record name | cis-Cyclobutane-1,3-dicarboxylic acid | |
Source | EPA DSSTox | |
URL | https://comptox.epa.gov/dashboard/DTXSID90178702 | |
Description | DSSTox provides a high quality public chemistry resource for supporting improved predictive toxicology. | |
Record name | rac-(1s,3s)-cyclobutane-1,3-dicarboxylic acid | |
Source | European Chemicals Agency (ECHA) | |
URL | https://echa.europa.eu/information-on-chemicals | |
Description | The European Chemicals Agency (ECHA) is an agency of the European Union which is the driving force among regulatory authorities in implementing the EU's groundbreaking chemicals legislation for the benefit of human health and the environment as well as for innovation and competitiveness. | |
Explanation | Use of the information, documents and data from the ECHA website is subject to the terms and conditions of this Legal Notice, and subject to other binding limitations provided for under applicable law, the information, documents and data made available on the ECHA website may be reproduced, distributed and/or used, totally or in part, for non-commercial purposes provided that ECHA is acknowledged as the source: "Source: European Chemicals Agency, http://echa.europa.eu/". Such acknowledgement must be included in each copy of the material. ECHA permits and encourages organisations and individuals to create links to the ECHA website under the following cumulative conditions: Links can only be made to webpages that provide a link to the Legal Notice page. | |
Retrosynthesis Analysis
AI-Powered Synthesis Planning: Our tool employs the Template_relevance Pistachio, Template_relevance Bkms_metabolic, Template_relevance Pistachio_ringbreaker, Template_relevance Reaxys, Template_relevance Reaxys_biocatalysis model, leveraging a vast database of chemical reactions to predict feasible synthetic routes.
One-Step Synthesis Focus: Specifically designed for one-step synthesis, it provides concise and direct routes for your target compounds, streamlining the synthesis process.
Accurate Predictions: Utilizing the extensive PISTACHIO, BKMS_METABOLIC, PISTACHIO_RINGBREAKER, REAXYS, REAXYS_BIOCATALYSIS database, our tool offers high-accuracy predictions, reflecting the latest in chemical research and data.
Strategy Settings
Precursor scoring | Relevance Heuristic |
---|---|
Min. plausibility | 0.01 |
Model | Template_relevance |
Template Set | Pistachio/Bkms_metabolic/Pistachio_ringbreaker/Reaxys/Reaxys_biocatalysis |
Top-N result to add to graph | 6 |
Feasible Synthetic Routes
Disclaimer and Information on In-Vitro Research Products
Please be aware that all articles and product information presented on BenchChem are intended solely for informational purposes. The products available for purchase on BenchChem are specifically designed for in-vitro studies, which are conducted outside of living organisms. In-vitro studies, derived from the Latin term "in glass," involve experiments performed in controlled laboratory settings using cells or tissues. It is important to note that these products are not categorized as medicines or drugs, and they have not received approval from the FDA for the prevention, treatment, or cure of any medical condition, ailment, or disease. We must emphasize that any form of bodily introduction of these products into humans or animals is strictly prohibited by law. It is essential to adhere to these guidelines to ensure compliance with legal and ethical standards in research and experimentation.