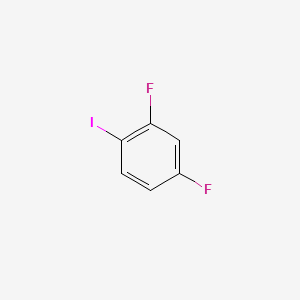
2,4-Difluoroiodobenzene
Overview
Description
Synthesis Analysis
The synthesis of complex fluorinated aromatic compounds can be achieved through various methods, including aromatic nucleophilic substitution. For instance, the synthesis of 1,4-bis(dimesitylphosphino)-2,3,5,6-tetrafluorobenzene was accomplished by reacting lithium dimesitylphosphide with hexafluorobenzene . This method could potentially be adapted for the synthesis of 2,4-difluoroiodobenzene by choosing appropriate starting materials and reaction conditions.
Molecular Structure Analysis
The molecular structure of fluorinated aromatic compounds can be quite intricate due to the presence of bulky substituents and the electronic effects of fluorine atoms. In the case of the synthesized tetrafluorobenzene derivatives, X-ray crystallography revealed large bond angles around the phosphorus atoms, indicating steric hindrance . This suggests that the molecular structure of 2,4-difluoroiodobenzene would also be influenced by the presence of fluorine atoms and any additional substituents.
Chemical Reactions Analysis
Fluorinated compounds often participate in unique chemical reactions due to the high electronegativity of fluorine. For example, the gem-difluorocyclobutane core, a valuable structural element in medicinal chemistry, can be synthesized through a migratory gem-difluorination of aryl-substituted methylenecyclopropanes, which involves a Wagner-Meerwein rearrangement . This highlights the reactivity of fluorinated compounds and suggests that 2,4-difluoroiodobenzene could undergo similar interesting transformations.
Physical and Chemical Properties Analysis
The physical and chemical properties of fluorinated aromatic compounds are often characterized by their reactivity and stability. The electrochemical properties of such compounds can be studied through measurements that reveal their redox behavior . Additionally, the crystallization of related compounds from solvents like chlorobenzene can result in the formation of solvates, which can affect the properties of the material . These findings imply that 2,4-difluoroiodobenzene would have distinct physical and chemical properties that could be explored through similar analytical techniques.
Scientific Research Applications
1. Gas-Phase Structural Isomer Identification
In a study by Burt et al. (2018), 2,4-difluoroiodobenzene was used to identify the gas-phase structures of difluoroiodobenzene isomers. This was achieved through Coulomb explosion of aligned molecules, utilizing femtosecond laser-induced Coulomb explosion and laser-induced adiabatic alignment techniques. This research demonstrates the application of 2,4-difluoroiodobenzene in advanced molecular structure identification methods (Burt et al., 2018).
2. Fragmentation Dynamics Study
Ablikim et al. (2017) explored the fragmentation dynamics of 2,4-difluoroiodobenzene following iodine 4d inner-shell photoionization. The research provided insights into different fragmentation mechanisms and timescales, distinguishing between sequential fragmentation and immediate breakup of molecules. This study underlines the use of 2,4-difluoroiodobenzene in understanding molecular fragmentation processes (Ablikim et al., 2017).
3. Laser-Induced 3D Alignment and Orientation
Nevo et al. (2009) conducted a study on the laser-induced 3D alignment and orientation of 2,4-difluoroiodobenzene molecules. The research utilized a strong inhomogeneous static electric field for spatial dispersion and rotational state selection, followed by laser-induced alignment and orientation. This highlights the role of 2,4-difluoroiodobenzene in advanced molecular alignment and orientation studies (Nevo et al., 2009).
4. Synthesis of Highly Substituted Oxazoles
Saito et al. (2012) utilized 2,4-difluoroiodobenzene in the synthesis of highly substituted oxazoles. The research demonstrated an efficient method for reacting dicarbonyl and monocarbonyl compounds with nitriles, highlighting the compound's application in organic synthesis (Saito et al., 2012).
Safety and Hazards
Future Directions
properties
IUPAC Name |
2,4-difluoro-1-iodobenzene | |
---|---|---|
Source | PubChem | |
URL | https://pubchem.ncbi.nlm.nih.gov | |
Description | Data deposited in or computed by PubChem | |
InChI |
InChI=1S/C6H3F2I/c7-4-1-2-6(9)5(8)3-4/h1-3H | |
Source | PubChem | |
URL | https://pubchem.ncbi.nlm.nih.gov | |
Description | Data deposited in or computed by PubChem | |
InChI Key |
YKLDMAPEGQYZRT-UHFFFAOYSA-N | |
Source | PubChem | |
URL | https://pubchem.ncbi.nlm.nih.gov | |
Description | Data deposited in or computed by PubChem | |
Canonical SMILES |
C1=CC(=C(C=C1F)F)I | |
Source | PubChem | |
URL | https://pubchem.ncbi.nlm.nih.gov | |
Description | Data deposited in or computed by PubChem | |
Molecular Formula |
C6H3F2I | |
Source | PubChem | |
URL | https://pubchem.ncbi.nlm.nih.gov | |
Description | Data deposited in or computed by PubChem | |
DSSTOX Substance ID |
DTXSID70177192 | |
Record name | 1,3-Difluoro-4-iodobenzene | |
Source | EPA DSSTox | |
URL | https://comptox.epa.gov/dashboard/DTXSID70177192 | |
Description | DSSTox provides a high quality public chemistry resource for supporting improved predictive toxicology. | |
Molecular Weight |
239.99 g/mol | |
Source | PubChem | |
URL | https://pubchem.ncbi.nlm.nih.gov | |
Description | Data deposited in or computed by PubChem | |
CAS RN |
2265-93-2 | |
Record name | 1,3-Difluoro-4-iodobenzene | |
Source | ChemIDplus | |
URL | https://pubchem.ncbi.nlm.nih.gov/substance/?source=chemidplus&sourceid=0002265932 | |
Description | ChemIDplus is a free, web search system that provides access to the structure and nomenclature authority files used for the identification of chemical substances cited in National Library of Medicine (NLM) databases, including the TOXNET system. | |
Record name | 2265-93-2 | |
Source | DTP/NCI | |
URL | https://dtp.cancer.gov/dtpstandard/servlet/dwindex?searchtype=NSC&outputformat=html&searchlist=68264 | |
Description | The NCI Development Therapeutics Program (DTP) provides services and resources to the academic and private-sector research communities worldwide to facilitate the discovery and development of new cancer therapeutic agents. | |
Explanation | Unless otherwise indicated, all text within NCI products is free of copyright and may be reused without our permission. Credit the National Cancer Institute as the source. | |
Record name | 1,3-Difluoro-4-iodobenzene | |
Source | EPA DSSTox | |
URL | https://comptox.epa.gov/dashboard/DTXSID70177192 | |
Description | DSSTox provides a high quality public chemistry resource for supporting improved predictive toxicology. | |
Record name | 2,4-difluoro-1-iodobenzene | |
Source | European Chemicals Agency (ECHA) | |
URL | https://echa.europa.eu/information-on-chemicals | |
Description | The European Chemicals Agency (ECHA) is an agency of the European Union which is the driving force among regulatory authorities in implementing the EU's groundbreaking chemicals legislation for the benefit of human health and the environment as well as for innovation and competitiveness. | |
Explanation | Use of the information, documents and data from the ECHA website is subject to the terms and conditions of this Legal Notice, and subject to other binding limitations provided for under applicable law, the information, documents and data made available on the ECHA website may be reproduced, distributed and/or used, totally or in part, for non-commercial purposes provided that ECHA is acknowledged as the source: "Source: European Chemicals Agency, http://echa.europa.eu/". Such acknowledgement must be included in each copy of the material. ECHA permits and encourages organisations and individuals to create links to the ECHA website under the following cumulative conditions: Links can only be made to webpages that provide a link to the Legal Notice page. | |
Retrosynthesis Analysis
AI-Powered Synthesis Planning: Our tool employs the Template_relevance Pistachio, Template_relevance Bkms_metabolic, Template_relevance Pistachio_ringbreaker, Template_relevance Reaxys, Template_relevance Reaxys_biocatalysis model, leveraging a vast database of chemical reactions to predict feasible synthetic routes.
One-Step Synthesis Focus: Specifically designed for one-step synthesis, it provides concise and direct routes for your target compounds, streamlining the synthesis process.
Accurate Predictions: Utilizing the extensive PISTACHIO, BKMS_METABOLIC, PISTACHIO_RINGBREAKER, REAXYS, REAXYS_BIOCATALYSIS database, our tool offers high-accuracy predictions, reflecting the latest in chemical research and data.
Strategy Settings
Precursor scoring | Relevance Heuristic |
---|---|
Min. plausibility | 0.01 |
Model | Template_relevance |
Template Set | Pistachio/Bkms_metabolic/Pistachio_ringbreaker/Reaxys/Reaxys_biocatalysis |
Top-N result to add to graph | 6 |
Feasible Synthetic Routes
Q & A
Q1: What makes 2,4-Difluoroiodobenzene useful for N-arylation reactions?
A1: While the provided abstracts do not delve into specific advantages of 2,4-Difluoroiodobenzene, its structure suggests potential benefits. The presence of two fluorine atoms, being highly electronegative, can make the iodine atom more electrophilic, potentially increasing its reactivity in coupling reactions with nitrogen heterocycles [, ]. Additionally, the fluorine atoms can influence the molecule's electronic properties, potentially impacting the regioselectivity of the arylation reaction. Further research is needed to confirm these hypotheses and fully characterize the reactivity and selectivity of 2,4-Difluoroiodobenzene in N-arylation reactions.
Q2: Are there any spectroscopic data available for 2,4-Difluoroiodobenzene?
A2: Unfortunately, the provided abstracts [, ] do not offer specific spectroscopic data for 2,4-Difluoroiodobenzene. To obtain such information (NMR, IR, Mass Spectrometry, etc.), one would need to consult the full research articles or relevant chemical databases.
Disclaimer and Information on In-Vitro Research Products
Please be aware that all articles and product information presented on BenchChem are intended solely for informational purposes. The products available for purchase on BenchChem are specifically designed for in-vitro studies, which are conducted outside of living organisms. In-vitro studies, derived from the Latin term "in glass," involve experiments performed in controlled laboratory settings using cells or tissues. It is important to note that these products are not categorized as medicines or drugs, and they have not received approval from the FDA for the prevention, treatment, or cure of any medical condition, ailment, or disease. We must emphasize that any form of bodily introduction of these products into humans or animals is strictly prohibited by law. It is essential to adhere to these guidelines to ensure compliance with legal and ethical standards in research and experimentation.