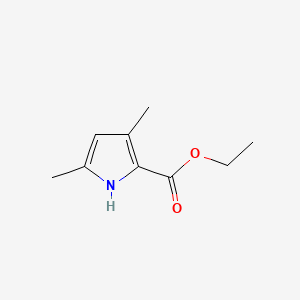
Ethyl 3,5-dimethyl-1H-pyrrole-2-carboxylate
Overview
Description
Ethyl 3,5-dimethyl-1H-pyrrole-2-carboxylate is a heterocyclic organic compound belonging to the pyrrole family. It is characterized by a pyrrole ring substituted with ethyl, methyl, and carboxylate groups. This compound is known for its stability and unique aromatic properties, making it valuable in various chemical and industrial applications .
Preparation Methods
Synthetic Routes and Reaction Conditions
Ethyl 3,5-dimethyl-1H-pyrrole-2-carboxylate can be synthesized through the reaction of ethyl cyanoacetate and acetylacetone. The reaction typically involves the use of a base, such as sodium ethoxide, in an ethanol solvent. The mixture is heated under reflux conditions to facilitate the formation of the pyrrole ring .
Industrial Production Methods
In industrial settings, the production of this compound follows similar synthetic routes but on a larger scale. The process involves the use of continuous flow reactors to ensure efficient mixing and reaction control. The product is then purified through distillation or recrystallization to achieve the desired purity levels .
Chemical Reactions Analysis
Types of Reactions
Ethyl 3,5-dimethyl-1H-pyrrole-2-carboxylate undergoes various chemical reactions, including:
Oxidation: The compound can be oxidized to form corresponding pyrrole-2-carboxylic acids.
Reduction: Reduction reactions can convert the carboxylate group to an alcohol or aldehyde.
Substitution: The methyl groups on the pyrrole ring can be substituted with other functional groups through electrophilic substitution reactions
Common Reagents and Conditions
Oxidation: Common oxidizing agents include potassium permanganate and chromium trioxide.
Reduction: Reducing agents such as lithium aluminum hydride or sodium borohydride are used.
Substitution: Electrophilic reagents like bromine or chlorine can be used under acidic conditions
Major Products Formed
Oxidation: Pyrrole-2-carboxylic acids.
Reduction: Pyrrole-2-carboxyl alcohols or aldehydes.
Substitution: Various substituted pyrrole derivatives depending on the electrophile used
Scientific Research Applications
Ethyl 3,5-dimethyl-1H-pyrrole-2-carboxylate has several scientific research applications:
Chemistry: Used as an intermediate in the synthesis of more complex heterocyclic compounds.
Biology: Investigated for its potential biological activities, including antimicrobial and antiviral properties.
Medicine: Explored for its potential use in drug development due to its unique structural properties.
Industry: Utilized in the production of dyes, pigments, and other fine chemicals
Mechanism of Action
The mechanism of action of ethyl 3,5-dimethyl-1H-pyrrole-2-carboxylate involves its interaction with various molecular targets. The compound’s aromatic structure allows it to participate in π-π interactions with biological molecules, potentially affecting enzyme activity and cellular processes. The exact pathways and targets are still under investigation, but its structural similarity to other bioactive pyrrole derivatives suggests potential interactions with nucleic acids and proteins .
Comparison with Similar Compounds
Ethyl 3,5-dimethyl-1H-pyrrole-2-carboxylate can be compared with similar compounds such as:
Ethyl 4-formyl-3,5-dimethyl-1H-pyrrole-2-carboxylate: This compound has a formyl group instead of a carboxylate group, leading to different reactivity and applications.
Ethyl 3,5-dimethyl-4-ethyl-1H-pyrrole-2-carboxylate: The presence of an additional ethyl group alters its physical and chemical properties.
The uniqueness of this compound lies in its specific substitution pattern, which imparts distinct reactivity and stability compared to its analogs.
Biological Activity
Ethyl 3,5-dimethyl-1H-pyrrole-2-carboxylate (EDPC) is a pyrrole derivative that has garnered attention for its potential biological activities, particularly in the fields of antimicrobial and anticancer research. This article explores the compound's biological activity, including its mechanisms, effectiveness against various pathogens, and its role as a precursor in the synthesis of more complex molecules.
Chemical Structure and Properties
This compound has the following chemical characteristics:
- Molecular Formula : C₉H₁₃N₁O₂
- Molecular Weight : 167.21 g/mol
- IUPAC Name : this compound
- SMILES Notation : CCOC(=O)C1=C(C=C(N1)C)C
The compound features a five-membered nitrogen-containing heterocyclic ring, which is a common structural motif in many biologically active molecules.
Antimicrobial Properties
Recent studies have demonstrated that EDPC exhibits significant antibacterial activity, particularly against Gram-positive bacteria. For instance, azomethines derived from EDPC have shown effectiveness against various bacterial strains. The antibacterial properties are attributed to the ability of these compounds to disrupt bacterial cell wall synthesis and interfere with metabolic pathways essential for bacterial survival .
Bacterial Strain | Activity |
---|---|
Staphylococcus aureus | Effective |
Bacillus subtilis | Effective |
Escherichia coli | Limited |
Anticancer Activity
EDPC has also been investigated for its potential as an anticancer agent. Research indicates that it can serve as a precursor for synthesizing more complex compounds with enhanced antitumor properties. For example, derivatives synthesized from EDPC have been tested against human carcinoma cell lines such as A-431 and A-549, showing promising inhibitory effects on cell proliferation .
In one study, several new compounds derived from EDPC were designed and synthesized, leading to significant cytotoxicity against cancer cells with IC50 values ranging from 0.065 to 9.4 µmol/L . This suggests that modifications to the pyrrole structure can enhance its biological activity.
The mechanisms underlying the biological activities of EDPC are still under investigation. However, it is hypothesized that:
- Antibacterial Mechanism : The compound may inhibit bacterial growth by disrupting cell wall synthesis or interfering with protein synthesis due to its structural similarity to amino acids involved in these processes.
- Anticancer Mechanism : The ability of EDPC-derived compounds to induce apoptosis in cancer cells may involve the activation of specific signaling pathways related to cell cycle regulation and programmed cell death.
Case Study 1: Antibacterial Efficacy
In a study published in Heterocyclic Communications, researchers synthesized various azomethines from EDPC and tested their antibacterial efficacy. The results indicated that certain derivatives exhibited higher antibacterial activity than EDPC itself, particularly against Gram-positive bacteria .
Case Study 2: Anticancer Activity
A series of pyrrole derivatives synthesized from EDPC were evaluated for their anticancer properties in vitro. The study demonstrated that specific modifications led to compounds with enhanced cytotoxic effects on multiple cancer cell lines, indicating a promising avenue for drug development .
Properties
IUPAC Name |
ethyl 3,5-dimethyl-1H-pyrrole-2-carboxylate | |
---|---|---|
Source | PubChem | |
URL | https://pubchem.ncbi.nlm.nih.gov | |
Description | Data deposited in or computed by PubChem | |
InChI |
InChI=1S/C9H13NO2/c1-4-12-9(11)8-6(2)5-7(3)10-8/h5,10H,4H2,1-3H3 | |
Source | PubChem | |
URL | https://pubchem.ncbi.nlm.nih.gov | |
Description | Data deposited in or computed by PubChem | |
InChI Key |
IZSBSZYFPYIJDI-UHFFFAOYSA-N | |
Source | PubChem | |
URL | https://pubchem.ncbi.nlm.nih.gov | |
Description | Data deposited in or computed by PubChem | |
Canonical SMILES |
CCOC(=O)C1=C(C=C(N1)C)C | |
Source | PubChem | |
URL | https://pubchem.ncbi.nlm.nih.gov | |
Description | Data deposited in or computed by PubChem | |
Molecular Formula |
C9H13NO2 | |
Source | PubChem | |
URL | https://pubchem.ncbi.nlm.nih.gov | |
Description | Data deposited in or computed by PubChem | |
DSSTOX Substance ID |
DTXSID40176410 | |
Record name | 1H-Pyrrole-2-carboxylic acid, 3,5-dimethyl-, ethyl ester | |
Source | EPA DSSTox | |
URL | https://comptox.epa.gov/dashboard/DTXSID40176410 | |
Description | DSSTox provides a high quality public chemistry resource for supporting improved predictive toxicology. | |
Molecular Weight |
167.20 g/mol | |
Source | PubChem | |
URL | https://pubchem.ncbi.nlm.nih.gov | |
Description | Data deposited in or computed by PubChem | |
CAS No. |
2199-44-2 | |
Record name | 1H-Pyrrole-2-carboxylic acid, 3,5-dimethyl-, ethyl ester | |
Source | ChemIDplus | |
URL | https://pubchem.ncbi.nlm.nih.gov/substance/?source=chemidplus&sourceid=0002199442 | |
Description | ChemIDplus is a free, web search system that provides access to the structure and nomenclature authority files used for the identification of chemical substances cited in National Library of Medicine (NLM) databases, including the TOXNET system. | |
Record name | 2199-44-2 | |
Source | DTP/NCI | |
URL | https://dtp.cancer.gov/dtpstandard/servlet/dwindex?searchtype=NSC&outputformat=html&searchlist=121772 | |
Description | The NCI Development Therapeutics Program (DTP) provides services and resources to the academic and private-sector research communities worldwide to facilitate the discovery and development of new cancer therapeutic agents. | |
Explanation | Unless otherwise indicated, all text within NCI products is free of copyright and may be reused without our permission. Credit the National Cancer Institute as the source. | |
Record name | 2199-44-2 | |
Source | DTP/NCI | |
URL | https://dtp.cancer.gov/dtpstandard/servlet/dwindex?searchtype=NSC&outputformat=html&searchlist=10763 | |
Description | The NCI Development Therapeutics Program (DTP) provides services and resources to the academic and private-sector research communities worldwide to facilitate the discovery and development of new cancer therapeutic agents. | |
Explanation | Unless otherwise indicated, all text within NCI products is free of copyright and may be reused without our permission. Credit the National Cancer Institute as the source. | |
Record name | 1H-Pyrrole-2-carboxylic acid, 3,5-dimethyl-, ethyl ester | |
Source | EPA DSSTox | |
URL | https://comptox.epa.gov/dashboard/DTXSID40176410 | |
Description | DSSTox provides a high quality public chemistry resource for supporting improved predictive toxicology. | |
Record name | 3,5-Dimethyl-1H-pyrrole-2-carboxylic acid ethyl ester | |
Source | European Chemicals Agency (ECHA) | |
URL | https://echa.europa.eu/information-on-chemicals | |
Description | The European Chemicals Agency (ECHA) is an agency of the European Union which is the driving force among regulatory authorities in implementing the EU's groundbreaking chemicals legislation for the benefit of human health and the environment as well as for innovation and competitiveness. | |
Explanation | Use of the information, documents and data from the ECHA website is subject to the terms and conditions of this Legal Notice, and subject to other binding limitations provided for under applicable law, the information, documents and data made available on the ECHA website may be reproduced, distributed and/or used, totally or in part, for non-commercial purposes provided that ECHA is acknowledged as the source: "Source: European Chemicals Agency, http://echa.europa.eu/". Such acknowledgement must be included in each copy of the material. ECHA permits and encourages organisations and individuals to create links to the ECHA website under the following cumulative conditions: Links can only be made to webpages that provide a link to the Legal Notice page. | |
Retrosynthesis Analysis
AI-Powered Synthesis Planning: Our tool employs the Template_relevance Pistachio, Template_relevance Bkms_metabolic, Template_relevance Pistachio_ringbreaker, Template_relevance Reaxys, Template_relevance Reaxys_biocatalysis model, leveraging a vast database of chemical reactions to predict feasible synthetic routes.
One-Step Synthesis Focus: Specifically designed for one-step synthesis, it provides concise and direct routes for your target compounds, streamlining the synthesis process.
Accurate Predictions: Utilizing the extensive PISTACHIO, BKMS_METABOLIC, PISTACHIO_RINGBREAKER, REAXYS, REAXYS_BIOCATALYSIS database, our tool offers high-accuracy predictions, reflecting the latest in chemical research and data.
Strategy Settings
Precursor scoring | Relevance Heuristic |
---|---|
Min. plausibility | 0.01 |
Model | Template_relevance |
Template Set | Pistachio/Bkms_metabolic/Pistachio_ringbreaker/Reaxys/Reaxys_biocatalysis |
Top-N result to add to graph | 6 |
Feasible Synthetic Routes
Disclaimer and Information on In-Vitro Research Products
Please be aware that all articles and product information presented on BenchChem are intended solely for informational purposes. The products available for purchase on BenchChem are specifically designed for in-vitro studies, which are conducted outside of living organisms. In-vitro studies, derived from the Latin term "in glass," involve experiments performed in controlled laboratory settings using cells or tissues. It is important to note that these products are not categorized as medicines or drugs, and they have not received approval from the FDA for the prevention, treatment, or cure of any medical condition, ailment, or disease. We must emphasize that any form of bodily introduction of these products into humans or animals is strictly prohibited by law. It is essential to adhere to these guidelines to ensure compliance with legal and ethical standards in research and experimentation.