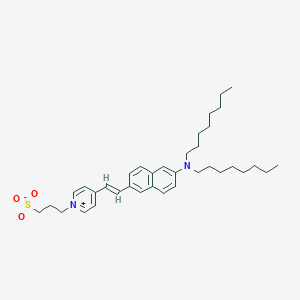
Di-8-ANEPPS
Overview
Description
These dyes are sensitive to changes in membrane potential and are widely used in the study of excitable cells, such as neurons and cardiac cells . Di-8-ANEPPS is particularly valued for its ability to detect submillisecond changes in membrane potential, making it a crucial tool in electrophysiological studies .
Mechanism of Action
Target of Action
Di-8-ANEPPS is a naphthylstyryl voltage-sensitive dye . Its primary targets are the cellular membranes where it detects changes in membrane potential . It has been used to monitor ion currents in pituitary GH3 cells .
Mode of Action
This compound operates by means of a change in its electronic structure, and consequently its fluorescence properties, in response to a change in the surrounding electric field . It has been shown to reversibly increase the amplitude of Ca2±activated K+ current . It enhances the activity of large-conductance Ca2±activated K+ (BKCa) channels .
Biochemical Pathways
The compound’s action primarily affects the membrane potential of cells . It is involved in physiological processes such as nerve-impulse propagation, muscle contraction, cell signaling, and ion-channel gating .
Pharmacokinetics
It is known that the dye is soluble in ethanol, dmso, and dmf . It is introduced into cells by direct addition of stock solution to cell culture medium, by using Pluronic™ F-127, or by retrograde labeling .
Result of Action
This compound has been used to detect transient (millisecond) potential changes in excitable cells, including single neurons, cardiac cells, and intact brains . It has been shown to decrease the firing of action potentials in GH3 cells .
Action Environment
The action of this compound is influenced by the environment. For instance, it is less susceptible to internalization than di-4-ANEPPS, permitting extended observation . Its spectral properties are highly dependent on the environment .
Biochemical Analysis
Biochemical Properties
Di-8-ANEPPS operates by means of a change in their electronic structure, and consequently their fluorescence properties, in response to a change in the surrounding electric field . This optical response is sufficiently fast to detect transient (millisecond) potential changes in excitable cells, including single neurons, cardiac cells, and intact brains . The magnitude of their potential-dependent fluorescence change is often small .
Cellular Effects
This compound has been shown to interact with the lipid bilayer of cell membranes . It has been used to measure transmembrane potentials statically and dynamically . The dye is less susceptible to internalization than di-4-ANEPPS, permitting extended observation .
Molecular Mechanism
This compound operates by means of a change in their electronic structure, and consequently their fluorescence properties, in response to a change in the surrounding electric field . These dyes display a potential-dependent shift in their excitation spectra, thus permitting the quantitation of membrane potential using excitation ratio measurements .
Temporal Effects in Laboratory Settings
This compound has been shown to be less susceptible to internalization than di-4-ANEPPS, permitting extended observation This suggests that this compound may be more stable over time in laboratory settings
Transport and Distribution
This compound is introduced into cells by direct addition of stock solution to cell culture medium . It is soluble in ethanol, DMSO, and DMF
Subcellular Localization
Preparation Methods
Synthetic Routes and Reaction Conditions: The synthesis of Di-8-ANEPPS involves the reaction of 4-(2-(6-(dioctylamino)-2-naphthyl)vinyl)pyridine with 1,3-propanesultone. The reaction is typically carried out in an organic solvent such as dimethyl sulfoxide (DMSO) or ethanol, under reflux conditions .
Industrial Production Methods: In industrial settings, the production of this compound follows similar synthetic routes but on a larger scale. The process involves the careful control of reaction conditions to ensure high yield and purity. The final product is usually purified through recrystallization or chromatography techniques .
Chemical Reactions Analysis
Types of Reactions: Di-8-ANEPPS primarily undergoes reactions related to its fluorescent properties. These include:
Fluorescence Quenching: Interaction with quenching agents can reduce its fluorescence intensity.
Photobleaching: Prolonged exposure to light can lead to the degradation of its fluorescent properties
Common Reagents and Conditions:
Solvents: Ethanol, DMSO, and dimethylformamide (DMF) are commonly used solvents.
Quenching Agents: Various ions and molecules can act as quenching agents, affecting the fluorescence of this compound
Major Products: The primary product of interest is the fluorescent this compound bound to cellular membranes, which is used for imaging and measuring membrane potential changes .
Scientific Research Applications
Di-8-ANEPPS has a wide range of applications in scientific research:
Chemistry: Used as a probe to study membrane dynamics and interactions.
Biology: Employed in live-cell imaging to monitor changes in membrane potential in neurons and cardiac cells.
Medicine: Utilized in research on neurological disorders and cardiac function.
Industry: Applied in the development of biosensors and diagnostic tools .
Comparison with Similar Compounds
Di-4-ANEPPS: Another member of the ANEP dye family, used for similar applications but with different spectral properties.
Di-2-ANEPEQ: A water-soluble ANEP dye, suitable for different experimental conditions
Uniqueness of Di-8-ANEPPS: this compound is unique due to its high lipophilicity, which allows it to remain in the outer leaflet of the cell membrane for extended periods. This property makes it particularly useful for long-term studies of membrane potential changes .
Biological Activity
Di-8-ANEPPS, a voltage-sensitive fluorescent dye, has garnered significant attention in biological research due to its utility in measuring transmembrane potentials across various cell types. This article delves into the biological activity of this compound, focusing on its mechanisms, applications, and recent findings from diverse studies.
Chemical Structure and Properties
This compound (1,1'-dioctadecyl-3,3,3',3'-tetramethylindocarbocyanine perchlorate) is characterized by a large dioctylamino tail that minimizes internalization and enhances its sensitivity for voltage measurements. The dye exhibits a unique twisted intramolecular charge transfer (TICT) mechanism that allows it to respond to changes in membrane potential effectively .
This compound operates primarily through fluorescence changes in response to alterations in membrane potential. The sensitivity of the dye is quantified as a change in relative fluorescence per unit change in membrane voltage, with reported values ranging from 0.015 to 0.15 (100 mV)⁻¹ depending on the measurement technique used .
Applications in Biological Research
This compound has been employed across various fields of biological research:
- Neuroscience : Used to measure action potentials in neurons, providing insights into neuronal signaling and excitability.
- Cardiology : Applied in cardiac myocytes to study electrical activity and arrhythmias .
- Cell Membrane Studies : Utilized to assess membrane fluidity and cholesterol content's impact on membrane dynamics .
Case Studies and Research Findings
Recent studies have highlighted the versatility of this compound in different biological contexts:
- Transmembrane Potential Measurement : In a study involving isolated myocytes, this compound effectively tracked voltage changes during action potentials, demonstrating its capability for real-time monitoring of electrical activity .
-
Cholesterol-Induced Rigidity : Research indicated that increasing cholesterol levels in model membranes significantly affected the fluorescence lifetime of this compound. Specifically, fluorescence lifetimes increased from 2.36 ns to 3.65 ns as cholesterol content rose from 0% to 50%, indicating reduced membrane fluidity .
Cholesterol Concentration Fluorescence Lifetime (ns) 0% 2.36 15% 2.70 24% 2.70 40% 3.52 50% 3.65 - Extracellular Vesicle Analysis : In studies examining extracellular vesicles (EVs), this compound was utilized for flow cytometry to quantify EVs' protein expression over time post-implantation . The dye's specificity allowed for detailed tracking of EV dynamics and immune responses.
Properties
IUPAC Name |
3-[4-[(E)-2-[6-(dioctylamino)naphthalen-2-yl]ethenyl]pyridin-1-ium-1-yl]propane-1-sulfonate | |
---|---|---|
Source | PubChem | |
URL | https://pubchem.ncbi.nlm.nih.gov | |
Description | Data deposited in or computed by PubChem | |
InChI |
InChI=1S/C36H52N2O3S/c1-3-5-7-9-11-13-25-38(26-14-12-10-8-6-4-2)36-21-20-34-30-33(18-19-35(34)31-36)17-16-32-22-27-37(28-23-32)24-15-29-42(39,40)41/h16-23,27-28,30-31H,3-15,24-26,29H2,1-2H3 | |
Source | PubChem | |
URL | https://pubchem.ncbi.nlm.nih.gov | |
Description | Data deposited in or computed by PubChem | |
InChI Key |
IXFSUSNUALIXLU-UHFFFAOYSA-N | |
Source | PubChem | |
URL | https://pubchem.ncbi.nlm.nih.gov | |
Description | Data deposited in or computed by PubChem | |
Canonical SMILES |
CCCCCCCCN(CCCCCCCC)C1=CC2=C(C=C1)C=C(C=C2)C=CC3=CC=[N+](C=C3)CCCS(=O)(=O)[O-] | |
Source | PubChem | |
URL | https://pubchem.ncbi.nlm.nih.gov | |
Description | Data deposited in or computed by PubChem | |
Isomeric SMILES |
CCCCCCCCN(CCCCCCCC)C1=CC2=C(C=C1)C=C(C=C2)/C=C/C3=CC=[N+](C=C3)CCCS(=O)(=O)[O-] | |
Source | PubChem | |
URL | https://pubchem.ncbi.nlm.nih.gov | |
Description | Data deposited in or computed by PubChem | |
Molecular Formula |
C36H52N2O3S | |
Source | PubChem | |
URL | https://pubchem.ncbi.nlm.nih.gov | |
Description | Data deposited in or computed by PubChem | |
DSSTOX Substance ID |
DTXSID40424766 | |
Record name | Di-8-ANEPPS | |
Source | EPA DSSTox | |
URL | https://comptox.epa.gov/dashboard/DTXSID40424766 | |
Description | DSSTox provides a high quality public chemistry resource for supporting improved predictive toxicology. | |
Molecular Weight |
592.9 g/mol | |
Source | PubChem | |
URL | https://pubchem.ncbi.nlm.nih.gov | |
Description | Data deposited in or computed by PubChem | |
CAS No. |
157134-53-7 | |
Record name | Di-8-ANEPPS | |
Source | EPA DSSTox | |
URL | https://comptox.epa.gov/dashboard/DTXSID40424766 | |
Description | DSSTox provides a high quality public chemistry resource for supporting improved predictive toxicology. | |
Retrosynthesis Analysis
AI-Powered Synthesis Planning: Our tool employs the Template_relevance Pistachio, Template_relevance Bkms_metabolic, Template_relevance Pistachio_ringbreaker, Template_relevance Reaxys, Template_relevance Reaxys_biocatalysis model, leveraging a vast database of chemical reactions to predict feasible synthetic routes.
One-Step Synthesis Focus: Specifically designed for one-step synthesis, it provides concise and direct routes for your target compounds, streamlining the synthesis process.
Accurate Predictions: Utilizing the extensive PISTACHIO, BKMS_METABOLIC, PISTACHIO_RINGBREAKER, REAXYS, REAXYS_BIOCATALYSIS database, our tool offers high-accuracy predictions, reflecting the latest in chemical research and data.
Strategy Settings
Precursor scoring | Relevance Heuristic |
---|---|
Min. plausibility | 0.01 |
Model | Template_relevance |
Template Set | Pistachio/Bkms_metabolic/Pistachio_ringbreaker/Reaxys/Reaxys_biocatalysis |
Top-N result to add to graph | 6 |
Feasible Synthetic Routes
Disclaimer and Information on In-Vitro Research Products
Please be aware that all articles and product information presented on BenchChem are intended solely for informational purposes. The products available for purchase on BenchChem are specifically designed for in-vitro studies, which are conducted outside of living organisms. In-vitro studies, derived from the Latin term "in glass," involve experiments performed in controlled laboratory settings using cells or tissues. It is important to note that these products are not categorized as medicines or drugs, and they have not received approval from the FDA for the prevention, treatment, or cure of any medical condition, ailment, or disease. We must emphasize that any form of bodily introduction of these products into humans or animals is strictly prohibited by law. It is essential to adhere to these guidelines to ensure compliance with legal and ethical standards in research and experimentation.