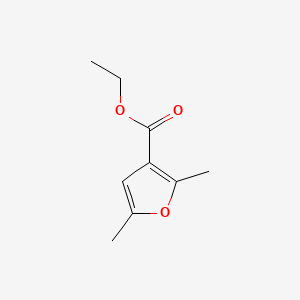
Ethyl 2,5-dimethylfuran-3-carboxylate
Overview
Description
Ethyl 2,5-dimethylfuran-3-carboxylate is a bio-based compound with the molecular formula C9H12O3 and a molecular weight of 168.19 g/mol.
Mechanism of Action
Target of Action
Ethyl 2,5-dimethylfuran-3-carboxylate is a furan platform chemical . Furan platform chemicals (FPCs) are derived from biomass and are used in the chemical industry as alternatives to traditional resources such as crude oil . .
Mode of Action
Furan derivatives are known for their reactivity, which makes them useful in the synthesis of new fuels and polymer precursors .
Biochemical Pathways
Furan derivatives are known to be involved in a variety of reactions, leading to the synthesis of a wide range of compounds .
Pharmacokinetics
It’s worth noting that the compound has a high gastrointestinal absorption and is bbb permeant .
Result of Action
As a furan derivative, it is likely involved in various chemical reactions, contributing to the synthesis of a wide range of compounds .
Action Environment
It’s worth noting that furan derivatives are part of a broader shift in the chemical industry towards the use of biomass as an alternative to traditional resources such as crude oil .
Biochemical Analysis
Biochemical Properties
Ethyl 2,5-dimethylfuran-3-carboxylate plays a significant role in biochemical reactions, particularly in the synthesis of other organic compounds. It interacts with various enzymes, proteins, and biomolecules. For instance, it is known to interact with cytochrome P450 enzymes, which are involved in the metabolism of many substances. The nature of these interactions often involves the oxidation of this compound, leading to the formation of more reactive intermediates .
Cellular Effects
This compound has been observed to influence various cellular processes. It affects cell signaling pathways, gene expression, and cellular metabolism. For example, it can modulate the activity of certain transcription factors, leading to changes in gene expression. Additionally, this compound can impact cellular metabolism by altering the activity of key metabolic enzymes .
Molecular Mechanism
The molecular mechanism of this compound involves its interaction with specific biomolecules. It can bind to enzymes, leading to either inhibition or activation of their activity. For instance, this compound has been shown to inhibit certain enzymes involved in the biosynthesis of fatty acids. This inhibition occurs through the binding of this compound to the active site of the enzyme, preventing the substrate from binding .
Temporal Effects in Laboratory Settings
In laboratory settings, the effects of this compound have been studied over time. It has been found to be relatively stable under standard laboratory conditions. Over extended periods, this compound can undergo degradation, leading to the formation of various by-products. Long-term studies have shown that this compound can have sustained effects on cellular function, particularly in terms of gene expression and enzyme activity .
Dosage Effects in Animal Models
The effects of this compound vary with different dosages in animal models. At low doses, it has been observed to have minimal impact on physiological functions. At higher doses, this compound can exhibit toxic effects, including liver and kidney damage. Threshold effects have been noted, where a certain dosage level leads to a significant increase in adverse effects.
Metabolic Pathways
This compound is involved in several metabolic pathways. It is primarily metabolized by cytochrome P450 enzymes, leading to the formation of various metabolites. These metabolites can further participate in biochemical reactions, affecting metabolic flux and metabolite levels. The interaction of this compound with cofactors such as NADPH is crucial for its metabolism .
Transport and Distribution
Within cells and tissues, this compound is transported and distributed through specific transporters and binding proteins. These transporters facilitate the movement of this compound across cellular membranes, ensuring its proper localization and accumulation. The distribution of this compound can influence its activity and function within different cellular compartments .
Subcellular Localization
This compound is localized in various subcellular compartments, including the cytoplasm and mitochondria. Its activity and function can be affected by its localization, as certain enzymes and biomolecules are compartment-specific. Post-translational modifications and targeting signals play a role in directing this compound to specific organelles, where it can exert its biochemical effects .
Preparation Methods
Ethyl 2,5-dimethylfuran-3-carboxylate can be synthesized through several methods. One common synthetic route involves the cyclization of (Z)-3-methylpent-2-en-4-yn-1-ol . Another method includes the reaction of ethyl 2-chloro-3-oxobutanoate with malonic acid dinitrile in the presence of sodium ethoxide . Industrial production methods often involve the use of biomass-derived furfural and 5-hydroxy-methylfurfural as starting materials .
Chemical Reactions Analysis
Ethyl 2,5-dimethylfuran-3-carboxylate undergoes various chemical reactions, including oxidation, reduction, and substitution. For example, it can react with triphenyltin chloride or diphenyltin dichloride in the presence of triethylamine to form organotin complexes . Another reaction involves the conversion of fructose into 2,5-dimethylfuran and ethyl levulinate in a cascade flow reactor . Common reagents used in these reactions include sodium hydroxide, ethanol, and sulfuric acid . Major products formed from these reactions include 2,5-dimethylfuran-3-carboxylic acid and its derivatives .
Scientific Research Applications
Ethyl 2,5-dimethylfuran-3-carboxylate has a wide range of scientific research applications. In chemistry, it is used as a building block for the synthesis of various heterocyclic compounds . In biology, it has been studied for its potential anticancer properties . In medicine, it is being explored for its potential use in drug development . In industry, it is used as a precursor for the production of bio-based polymers and other materials.
Comparison with Similar Compounds
Ethyl 2,5-dimethylfuran-3-carboxylate can be compared with other similar compounds, such as mthis compound and dimethyl 3,4-furandicarboxylate . Other similar compounds include ethyl 5-amino-4-cyano-2-methylfuran-3-carboxylate and ethyl 4-acetyl-5-methylfuran-3-carboxylate, which have been studied for their biological activities and synthetic applications .
Properties
IUPAC Name |
ethyl 2,5-dimethylfuran-3-carboxylate | |
---|---|---|
Source | PubChem | |
URL | https://pubchem.ncbi.nlm.nih.gov | |
Description | Data deposited in or computed by PubChem | |
InChI |
InChI=1S/C9H12O3/c1-4-11-9(10)8-5-6(2)12-7(8)3/h5H,4H2,1-3H3 | |
Source | PubChem | |
URL | https://pubchem.ncbi.nlm.nih.gov | |
Description | Data deposited in or computed by PubChem | |
InChI Key |
DNYLSCDHFCSDRM-UHFFFAOYSA-N | |
Source | PubChem | |
URL | https://pubchem.ncbi.nlm.nih.gov | |
Description | Data deposited in or computed by PubChem | |
Canonical SMILES |
CCOC(=O)C1=C(OC(=C1)C)C | |
Source | PubChem | |
URL | https://pubchem.ncbi.nlm.nih.gov | |
Description | Data deposited in or computed by PubChem | |
Molecular Formula |
C9H12O3 | |
Source | PubChem | |
URL | https://pubchem.ncbi.nlm.nih.gov | |
Description | Data deposited in or computed by PubChem | |
DSSTOX Substance ID |
DTXSID00183367 | |
Record name | 3-Furancarboxylic acid, 2,5-dimethyl-, ethyl ester | |
Source | EPA DSSTox | |
URL | https://comptox.epa.gov/dashboard/DTXSID00183367 | |
Description | DSSTox provides a high quality public chemistry resource for supporting improved predictive toxicology. | |
Molecular Weight |
168.19 g/mol | |
Source | PubChem | |
URL | https://pubchem.ncbi.nlm.nih.gov | |
Description | Data deposited in or computed by PubChem | |
CAS No. |
29113-63-1 | |
Record name | 3-Furancarboxylic acid, 2,5-dimethyl-, ethyl ester | |
Source | ChemIDplus | |
URL | https://pubchem.ncbi.nlm.nih.gov/substance/?source=chemidplus&sourceid=0029113631 | |
Description | ChemIDplus is a free, web search system that provides access to the structure and nomenclature authority files used for the identification of chemical substances cited in National Library of Medicine (NLM) databases, including the TOXNET system. | |
Record name | 3-Furancarboxylic acid, 2,5-dimethyl-, ethyl ester | |
Source | EPA DSSTox | |
URL | https://comptox.epa.gov/dashboard/DTXSID00183367 | |
Description | DSSTox provides a high quality public chemistry resource for supporting improved predictive toxicology. | |
Synthesis routes and methods
Procedure details
Retrosynthesis Analysis
AI-Powered Synthesis Planning: Our tool employs the Template_relevance Pistachio, Template_relevance Bkms_metabolic, Template_relevance Pistachio_ringbreaker, Template_relevance Reaxys, Template_relevance Reaxys_biocatalysis model, leveraging a vast database of chemical reactions to predict feasible synthetic routes.
One-Step Synthesis Focus: Specifically designed for one-step synthesis, it provides concise and direct routes for your target compounds, streamlining the synthesis process.
Accurate Predictions: Utilizing the extensive PISTACHIO, BKMS_METABOLIC, PISTACHIO_RINGBREAKER, REAXYS, REAXYS_BIOCATALYSIS database, our tool offers high-accuracy predictions, reflecting the latest in chemical research and data.
Strategy Settings
Precursor scoring | Relevance Heuristic |
---|---|
Min. plausibility | 0.01 |
Model | Template_relevance |
Template Set | Pistachio/Bkms_metabolic/Pistachio_ringbreaker/Reaxys/Reaxys_biocatalysis |
Top-N result to add to graph | 6 |
Feasible Synthetic Routes
Disclaimer and Information on In-Vitro Research Products
Please be aware that all articles and product information presented on BenchChem are intended solely for informational purposes. The products available for purchase on BenchChem are specifically designed for in-vitro studies, which are conducted outside of living organisms. In-vitro studies, derived from the Latin term "in glass," involve experiments performed in controlled laboratory settings using cells or tissues. It is important to note that these products are not categorized as medicines or drugs, and they have not received approval from the FDA for the prevention, treatment, or cure of any medical condition, ailment, or disease. We must emphasize that any form of bodily introduction of these products into humans or animals is strictly prohibited by law. It is essential to adhere to these guidelines to ensure compliance with legal and ethical standards in research and experimentation.