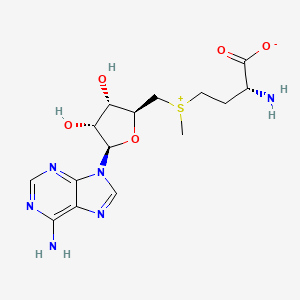
s-Adenosyl-d-methionine
- Click on QUICK INQUIRY to receive a quote from our team of experts.
- With the quality product at a COMPETITIVE price, you can focus more on your research.
Overview
Description
s-Adenosyl-d-methionine is a naturally occurring compound found in all living organisms. It plays a crucial role in various biochemical processes, including methylation, transsulfuration, and aminopropylation. This compound is synthesized from adenosine triphosphate (ATP) and methionine by the enzyme methionine adenosyltransferase . It is involved in the transfer of methyl groups to nucleic acids, proteins, lipids, and secondary metabolites .
Preparation Methods
Synthetic Routes and Reaction Conditions
s-Adenosyl-d-methionine can be synthesized enzymatically from ATP and methionine using methionine adenosyltransferase . The reaction conditions typically involve a buffered aqueous solution at a pH of around 7.5, with the presence of magnesium ions to stabilize the ATP . The enzyme catalyzes the transfer of the adenosyl group from ATP to methionine, forming this compound and releasing inorganic phosphate and pyrophosphate as by-products .
Industrial Production Methods
Industrial production of this compound often involves microbial fermentation using genetically engineered strains of yeast or bacteria . These microorganisms are optimized to overproduce methionine adenosyltransferase and to enhance the availability of ATP and methionine . The fermentation process is carried out in bioreactors under controlled conditions of temperature, pH, and aeration to maximize yield . After fermentation, the compound is purified using techniques such as ion exchange chromatography and crystallization .
Chemical Reactions Analysis
Types of Reactions
s-Adenosyl-d-methionine undergoes several types of chemical reactions, including:
Methylation: It donates a methyl group to various substrates, such as nucleic acids, proteins, and lipids.
Transsulfuration: It participates in the conversion of homocysteine to cysteine.
Aminopropylation: It is involved in the synthesis of polyamines, which are essential for cell growth and differentiation.
Common Reagents and Conditions
The methylation reactions involving this compound typically require the presence of methyltransferase enzymes and occur under physiological conditions (pH 7.4, 37°C) . Transsulfuration reactions involve enzymes such as cystathionine beta-synthase and cystathionine gamma-lyase . Aminopropylation reactions are catalyzed by enzymes like spermidine synthase and spermine synthase .
Major Products
The major products formed from these reactions include methylated nucleic acids and proteins, cysteine, and polyamines such as spermidine and spermine .
Scientific Research Applications
Neuropsychiatric Applications
Depression Treatment
SAMe has been extensively studied for its antidepressant effects. It is believed to enhance the methylation of neurotransmitters, which plays a significant role in mood regulation. Clinical trials have shown that SAMe can be effective in treating major depressive disorder, particularly in patients who do not respond to conventional treatments. For instance, a systematic review indicated that SAMe supplementation resulted in significant improvements in depression scores compared to placebo groups .
Mechanisms of Action
The mechanisms through which SAMe exerts its effects include:
- Transmethylation Pathways : SAMe acts as a methyl donor in the methylation of DNA, RNA, and proteins, influencing gene expression and neurotransmitter synthesis .
- Anti-inflammatory Effects : SAMe has been shown to reduce levels of pro-inflammatory cytokines, which are often elevated in depressive disorders .
Liver Health
Liver Disease Treatment
SAMe is utilized as a treatment for liver diseases such as cirrhosis and hepatitis. It aids in liver function by enhancing glutathione levels, a potent antioxidant that protects liver cells from damage. Studies have indicated that SAMe supplementation can improve liver function tests and reduce symptoms associated with liver disease .
Clinical Evidence
A review highlighted that patients with chronic liver disease experienced significant benefits from SAMe therapy, including improved biochemical markers of liver function and reduced symptoms of fatigue and malaise .
Joint Health
Osteoarthritis Management
SAMe is also marketed as a dietary supplement for osteoarthritis relief. Research suggests that it may alleviate pain and improve joint function comparable to non-steroidal anti-inflammatory drugs (NSAIDs) without the associated gastrointestinal side effects .
Clinical Trials
A systematic review found that SAMe was effective in reducing pain and improving physical function in osteoarthritis patients. In some studies, it was reported to be as effective as NSAIDs with fewer adverse effects .
Summary of Research Findings
The following table summarizes key findings from various studies on the applications of S-Adenosyl-d-methionine:
Case Studies
Several case studies have illustrated the effectiveness of SAMe in clinical settings:
- Case Study 1 : A patient with treatment-resistant depression showed marked improvement after adding SAMe to their regimen, achieving remission within weeks .
- Case Study 2 : In a cohort study involving patients with chronic liver disease, those receiving SAMe demonstrated better clinical outcomes compared to those who did not receive supplementation .
Mechanism of Action
s-Adenosyl-d-methionine exerts its effects by donating a methyl group to various substrates in a process called transmethylation . This process is catalyzed by methyltransferase enzymes and is essential for the regulation of gene expression, protein function, and lipid metabolism . The compound also participates in the transsulfuration pathway, which is crucial for the synthesis of cysteine and glutathione . Additionally, it is involved in the aminopropylation pathway, which is important for the production of polyamines .
Comparison with Similar Compounds
s-Adenosyl-d-methionine is unique in its ability to participate in multiple biochemical pathways, including methylation, transsulfuration, and aminopropylation . Similar compounds include:
Methionine: A precursor of this compound, involved in protein synthesis and methylation reactions.
Adenosine triphosphate (ATP): Provides the adenosyl group for the synthesis of this compound.
S-Adenosylhomocysteine (SAH): A by-product of methylation reactions involving this compound, which is further metabolized to homocysteine.
This compound stands out due to its versatility and involvement in various essential biochemical processes .
Properties
Molecular Formula |
C15H22N6O5S |
---|---|
Molecular Weight |
398.4 g/mol |
IUPAC Name |
(2R)-2-amino-4-[[(2S,3S,4R,5R)-5-(6-aminopurin-9-yl)-3,4-dihydroxyoxolan-2-yl]methyl-methylsulfonio]butanoate |
InChI |
InChI=1S/C15H22N6O5S/c1-27(3-2-7(16)15(24)25)4-8-10(22)11(23)14(26-8)21-6-20-9-12(17)18-5-19-13(9)21/h5-8,10-11,14,22-23H,2-4,16H2,1H3,(H2-,17,18,19,24,25)/t7-,8-,10-,11-,14-,27?/m1/s1 |
InChI Key |
MEFKEPWMEQBLKI-XCPQSEKJSA-N |
Isomeric SMILES |
C[S+](CC[C@H](C(=O)[O-])N)C[C@@H]1[C@H]([C@H]([C@@H](O1)N2C=NC3=C(N=CN=C32)N)O)O |
Canonical SMILES |
C[S+](CCC(C(=O)[O-])N)CC1C(C(C(O1)N2C=NC3=C(N=CN=C32)N)O)O |
Origin of Product |
United States |
Disclaimer and Information on In-Vitro Research Products
Please be aware that all articles and product information presented on BenchChem are intended solely for informational purposes. The products available for purchase on BenchChem are specifically designed for in-vitro studies, which are conducted outside of living organisms. In-vitro studies, derived from the Latin term "in glass," involve experiments performed in controlled laboratory settings using cells or tissues. It is important to note that these products are not categorized as medicines or drugs, and they have not received approval from the FDA for the prevention, treatment, or cure of any medical condition, ailment, or disease. We must emphasize that any form of bodily introduction of these products into humans or animals is strictly prohibited by law. It is essential to adhere to these guidelines to ensure compliance with legal and ethical standards in research and experimentation.