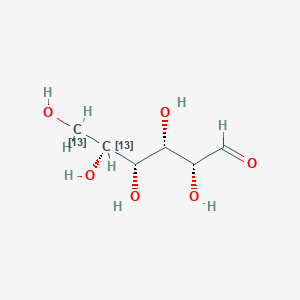
D-glucose-5,6-13C2
- Click on QUICK INQUIRY to receive a quote from our team of experts.
- With the quality product at a COMPETITIVE price, you can focus more on your research.
Overview
Description
D-glucose-5,6-13C2: is a stable isotope-labeled form of D-glucose, where the carbon atoms at positions 5 and 6 are replaced with the carbon-13 isotope. This compound is widely used in various scientific research fields due to its unique properties and applications.
Preparation Methods
Synthetic Routes and Reaction Conditions: The synthesis of D-glucose-5,6-13C2 typically involves the incorporation of carbon-13 into the glucose molecule. This can be achieved through various synthetic routes, including the use of labeled precursors in the biosynthesis of glucose. The reaction conditions often involve controlled environments to ensure the precise incorporation of the isotope.
Industrial Production Methods: Industrial production of this compound involves large-scale synthesis using labeled carbon sources. The process is optimized to achieve high yields and purity, often exceeding 99% isotopic purity . The production methods are designed to be cost-effective and scalable to meet the demands of research and industrial applications.
Chemical Reactions Analysis
Types of Reactions: D-glucose-5,6-13C2 undergoes various chemical reactions, including oxidation, reduction, and substitution. These reactions are essential for studying metabolic pathways and enzyme mechanisms.
Common Reagents and Conditions:
Oxidation: Common reagents include oxidizing agents like potassium permanganate and nitric acid. The reaction conditions typically involve aqueous solutions and controlled temperatures.
Reduction: Reducing agents such as sodium borohydride and hydrogen gas are used under mild conditions to reduce the glucose molecule.
Substitution: Substitution reactions often involve nucleophiles like halides or amines, with conditions tailored to facilitate the replacement of specific functional groups.
Major Products: The major products formed from these reactions include various glucose derivatives, which are used to study biochemical processes and metabolic pathways.
Scientific Research Applications
Chemistry: D-glucose-5,6-13C2 is used as a tracer in metabolic studies to investigate the pathways of glucose metabolism. It helps in understanding the dynamics of glucose utilization and storage in living organisms .
Biology: In biological research, this compound is used to study the role of glucose in cellular processes. It aids in the investigation of glucose transport, glycolysis, and other metabolic pathways .
Medicine: this compound is employed in medical research to study glucose metabolism in various diseases, including diabetes and cancer. It helps in understanding the alterations in glucose metabolism associated with these conditions .
Industry: In the industrial sector, this compound is used in the production of labeled pharmaceuticals and as a standard in analytical chemistry .
Mechanism of Action
D-glucose-5,6-13C2 exerts its effects by participating in metabolic pathways similar to natural glucose. The incorporation of carbon-13 allows for the tracking of glucose molecules through various biochemical processes. The molecular targets include enzymes involved in glycolysis, gluconeogenesis, and other metabolic pathways .
Comparison with Similar Compounds
- D-glucose-1,2-13C2
- D-glucose-2,5-13C2
- D-glucose-13C6
Uniqueness: D-glucose-5,6-13C2 is unique due to the specific labeling at positions 5 and 6, which allows for targeted studies of metabolic pathways involving these carbon atoms. This specificity makes it particularly valuable in research focused on the detailed mechanisms of glucose metabolism .
Biological Activity
D-Glucose-5,6-13C2 is a stable isotope-labeled form of glucose that has garnered attention in metabolic research due to its unique properties and applications in studying glucose metabolism. This article delves into the biological activity of this compound, highlighting its metabolic pathways, applications in clinical studies, and research findings.
Overview of this compound
This compound is a glucose molecule where the carbons at positions 5 and 6 are isotopically labeled with carbon-13. This labeling allows for the tracking of glucose metabolism through various biochemical pathways using techniques such as nuclear magnetic resonance (NMR) spectroscopy and mass spectrometry. The compound's structure is represented as follows:
Property | Details |
---|---|
Chemical Formula | C6H12O6 |
Molecular Weight | 180.18 g/mol |
CAS Number | 11600783 |
Metabolic Pathways
D-glucose serves as a primary energy source in living organisms. Upon ingestion, it undergoes several metabolic processes:
- Glycolysis : D-glucose is converted into pyruvate through a series of enzymatic reactions, producing ATP and NADH.
- Krebs Cycle (Citric Acid Cycle) : Pyruvate enters the mitochondria and is further oxidized to produce additional ATP, NADH, and FADH2.
- Pentose Phosphate Pathway : This pathway generates NADPH and ribose-5-phosphate for nucleotide synthesis.
The incorporation of 13C isotopes allows researchers to trace these pathways more effectively by analyzing the distribution of labeled carbon atoms in metabolic products.
In Vivo Studies
Research has demonstrated the utility of this compound in understanding cerebral metabolism. A study highlighted distinct metabolic behaviors in different brain regions when infused with [1,6-13C2]glucose:
- Hypothalamus : Exhibited higher glycolytic activity.
- Hippocampus : Relied more on oxidative metabolism .
Another study utilized hyperpolarized [2,3,4,6,6-2H5, 3,4-13C2]-D-glucose to enhance signal detection during NMR analysis, revealing more precise metabolic tracking capabilities compared to non-labeled glucose .
Clinical Applications
This compound has been employed in clinical settings to assess insulin sensitivity and glucose metabolism. A minimally invasive breath test using this compound demonstrated its potential for evaluating metabolic disorders such as type 2 diabetes. The test measures the oxidation of 13CO2 from the metabolized glucose to provide insights into glucose utilization .
Case Studies
-
Study on Glucose Metabolism in Type 2 Diabetes :
- Objective : To evaluate insulin resistance using this compound.
- Methodology : Participants underwent a breath test measuring CO2 production after ingesting labeled glucose.
- Findings : Significant differences in CO2 production rates were observed between healthy individuals and those with insulin resistance .
-
Neuroimaging Study :
- Objective : To investigate cerebral glucose metabolism using magnetic resonance spectroscopy (MRS).
- Methodology : Infusion of [1,6-13C2]-D-glucose allowed real-time monitoring of metabolic changes in the brain.
- Findings : The study revealed critical insights into how different brain regions metabolize glucose under various physiological conditions .
Properties
Molecular Formula |
C6H12O6 |
---|---|
Molecular Weight |
182.14 g/mol |
IUPAC Name |
(2R,3S,4R,5R)-2,3,4,5,6-pentahydroxy(5,6-13C2)hexanal |
InChI |
InChI=1S/C6H12O6/c7-1-3(9)5(11)6(12)4(10)2-8/h1,3-6,8-12H,2H2/t3-,4+,5+,6+/m0/s1/i2+1,4+1 |
InChI Key |
GZCGUPFRVQAUEE-TWXOKBJSSA-N |
Isomeric SMILES |
[13CH2]([13C@H]([C@H]([C@@H]([C@H](C=O)O)O)O)O)O |
Canonical SMILES |
C(C(C(C(C(C=O)O)O)O)O)O |
Origin of Product |
United States |
Disclaimer and Information on In-Vitro Research Products
Please be aware that all articles and product information presented on BenchChem are intended solely for informational purposes. The products available for purchase on BenchChem are specifically designed for in-vitro studies, which are conducted outside of living organisms. In-vitro studies, derived from the Latin term "in glass," involve experiments performed in controlled laboratory settings using cells or tissues. It is important to note that these products are not categorized as medicines or drugs, and they have not received approval from the FDA for the prevention, treatment, or cure of any medical condition, ailment, or disease. We must emphasize that any form of bodily introduction of these products into humans or animals is strictly prohibited by law. It is essential to adhere to these guidelines to ensure compliance with legal and ethical standards in research and experimentation.