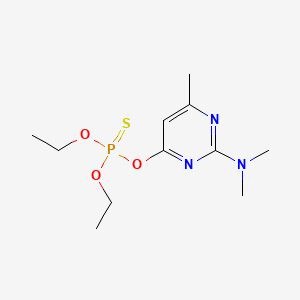
Pyrimitate
Overview
Description
Pyrimitate is a useful research compound. Its molecular formula is C11H20N3O3PS and its molecular weight is 305.34 g/mol. The purity is usually 95%.
BenchChem offers high-quality this compound suitable for many research applications. Different packaging options are available to accommodate customers' requirements. Please inquire for more information about this compound including the price, delivery time, and more detailed information at info@benchchem.com.
Mechanism of Action
Target of Action
Pyrimitate, also known as Diothyl, is a complex compound with a molecular formula of C11H20N3O3PS It’s structurally related to pyrimethamine, which is known to inhibit the dihydrofolate reductase of plasmodia, thereby blocking the biosynthesis of purines and pyrimidines essential for dna synthesis and cell multiplication .
Mode of Action
Pyrimethamine’s therapeutic action is based on the differential requirement between host and parasite for nucleic acid precursors involved in growth . This activity is highly selective against plasmodia and Toxoplasma gondii .
Biochemical Pathways
This compound may affect the de novo purine and pyrimidine biosynthesis pathways, similar to Pyrimethamine . These pathways are crucial for the synthesis of nucleic acids (RNA and DNA), which are essential for cell growth and multiplication . Pyrimethamine inhibits the dihydrofolate reductase enzyme, thereby blocking these pathways .
Pharmacokinetics
Pyrimethamine, a structurally related compound, is well-absorbed and has a protein binding of 87% . It is metabolized in the liver and excreted via the kidneys .
Result of Action
Based on its potential similarity to pyrimethamine, it may lead to the inhibition of dna synthesis and cell multiplication in certain parasites .
Biochemical Analysis
Biochemical Properties
Pyrimitate plays a significant role in biochemical reactions, particularly in its interaction with enzymes and proteins. It is known to inhibit acetylcholinesterase, an enzyme responsible for breaking down acetylcholine in the nervous system . This inhibition leads to an accumulation of acetylcholine, resulting in prolonged nerve signal transmission. This compound also interacts with other biomolecules, such as transport proteins, which facilitate its distribution within the organism.
Cellular Effects
This compound affects various types of cells and cellular processes. In neuronal cells, it disrupts normal cell function by inhibiting acetylcholinesterase, leading to excessive stimulation of acetylcholine receptors . This can cause symptoms such as muscle twitching, convulsions, and even paralysis. In non-neuronal cells, this compound can influence cell signaling pathways, gene expression, and cellular metabolism by interacting with key regulatory proteins and enzymes.
Molecular Mechanism
The molecular mechanism of this compound involves its binding to the active site of acetylcholinesterase, where it forms a covalent bond with the serine residue in the enzyme’s active site . This covalent modification prevents the enzyme from hydrolyzing acetylcholine, leading to an accumulation of the neurotransmitter in the synaptic cleft. Additionally, this compound may interact with other enzymes and proteins, altering their activity and affecting various cellular processes.
Temporal Effects in Laboratory Settings
In laboratory settings, the effects of this compound can change over time. The compound’s stability and degradation are influenced by factors such as temperature, pH, and exposure to light . Long-term studies have shown that this compound can have persistent effects on cellular function, including prolonged inhibition of acetylcholinesterase and disruption of normal cellular processes. These effects can be observed in both in vitro and in vivo studies.
Dosage Effects in Animal Models
The effects of this compound vary with different dosages in animal models. At low doses, this compound can cause mild symptoms such as muscle twitching and increased salivation . At higher doses, it can lead to severe toxicity, including convulsions, respiratory distress, and even death. Threshold effects have been observed, where a certain dosage level results in a significant increase in adverse effects. Toxicological studies have highlighted the importance of careful dosage management to avoid harmful outcomes.
Metabolic Pathways
This compound is involved in several metabolic pathways, including its biotransformation by liver enzymes . The compound is metabolized through processes such as oxidation, hydrolysis, and conjugation, leading to the formation of various metabolites. These metabolic pathways are crucial for the detoxification and elimination of this compound from the body. Enzymes such as cytochrome P450 play a key role in these metabolic processes.
Transport and Distribution
Within cells and tissues, this compound is transported and distributed by specific transporters and binding proteins . These proteins facilitate the movement of this compound across cell membranes and its accumulation in target tissues. The compound’s distribution is influenced by factors such as lipid solubility and binding affinity to transport proteins. Understanding the transport and distribution mechanisms of this compound is essential for predicting its effects on different tissues.
Subcellular Localization
This compound’s subcellular localization is determined by its interactions with cellular components and post-translational modifications . The compound can be directed to specific compartments or organelles, such as the endoplasmic reticulum or mitochondria, through targeting signals. These localization patterns can affect this compound’s activity and function within the cell. For example, its presence in the mitochondria may influence cellular energy metabolism and oxidative stress responses.
Properties
IUPAC Name |
4-diethoxyphosphinothioyloxy-N,N,6-trimethylpyrimidin-2-amine | |
---|---|---|
Source | PubChem | |
URL | https://pubchem.ncbi.nlm.nih.gov | |
Description | Data deposited in or computed by PubChem | |
InChI |
InChI=1S/C11H20N3O3PS/c1-6-15-18(19,16-7-2)17-10-8-9(3)12-11(13-10)14(4)5/h8H,6-7H2,1-5H3 | |
Source | PubChem | |
URL | https://pubchem.ncbi.nlm.nih.gov | |
Description | Data deposited in or computed by PubChem | |
InChI Key |
MLDVVJZNWASRQL-UHFFFAOYSA-N | |
Source | PubChem | |
URL | https://pubchem.ncbi.nlm.nih.gov | |
Description | Data deposited in or computed by PubChem | |
Canonical SMILES |
CCOP(=S)(OCC)OC1=NC(=NC(=C1)C)N(C)C | |
Source | PubChem | |
URL | https://pubchem.ncbi.nlm.nih.gov | |
Description | Data deposited in or computed by PubChem | |
Molecular Formula |
C11H20N3O3PS | |
Source | PubChem | |
URL | https://pubchem.ncbi.nlm.nih.gov | |
Description | Data deposited in or computed by PubChem | |
DSSTOX Substance ID |
DTXSID3058117 | |
Record name | Pyrimitate | |
Source | EPA DSSTox | |
URL | https://comptox.epa.gov/dashboard/DTXSID3058117 | |
Description | DSSTox provides a high quality public chemistry resource for supporting improved predictive toxicology. | |
Molecular Weight |
305.34 g/mol | |
Source | PubChem | |
URL | https://pubchem.ncbi.nlm.nih.gov | |
Description | Data deposited in or computed by PubChem | |
CAS No. |
5221-49-8 | |
Record name | Pyrimitate | |
Source | CAS Common Chemistry | |
URL | https://commonchemistry.cas.org/detail?cas_rn=5221-49-8 | |
Description | CAS Common Chemistry is an open community resource for accessing chemical information. Nearly 500,000 chemical substances from CAS REGISTRY cover areas of community interest, including common and frequently regulated chemicals, and those relevant to high school and undergraduate chemistry classes. This chemical information, curated by our expert scientists, is provided in alignment with our mission as a division of the American Chemical Society. | |
Explanation | The data from CAS Common Chemistry is provided under a CC-BY-NC 4.0 license, unless otherwise stated. | |
Record name | Pyrimitate [INN:BAN] | |
Source | ChemIDplus | |
URL | https://pubchem.ncbi.nlm.nih.gov/substance/?source=chemidplus&sourceid=0005221498 | |
Description | ChemIDplus is a free, web search system that provides access to the structure and nomenclature authority files used for the identification of chemical substances cited in National Library of Medicine (NLM) databases, including the TOXNET system. | |
Record name | Pyrimitate | |
Source | EPA DSSTox | |
URL | https://comptox.epa.gov/dashboard/DTXSID3058117 | |
Description | DSSTox provides a high quality public chemistry resource for supporting improved predictive toxicology. | |
Record name | Pyrimitate | |
Source | European Chemicals Agency (ECHA) | |
URL | https://echa.europa.eu/substance-information/-/substanceinfo/100.023.655 | |
Description | The European Chemicals Agency (ECHA) is an agency of the European Union which is the driving force among regulatory authorities in implementing the EU's groundbreaking chemicals legislation for the benefit of human health and the environment as well as for innovation and competitiveness. | |
Explanation | Use of the information, documents and data from the ECHA website is subject to the terms and conditions of this Legal Notice, and subject to other binding limitations provided for under applicable law, the information, documents and data made available on the ECHA website may be reproduced, distributed and/or used, totally or in part, for non-commercial purposes provided that ECHA is acknowledged as the source: "Source: European Chemicals Agency, http://echa.europa.eu/". Such acknowledgement must be included in each copy of the material. ECHA permits and encourages organisations and individuals to create links to the ECHA website under the following cumulative conditions: Links can only be made to webpages that provide a link to the Legal Notice page. | |
Record name | PYRIMITATE | |
Source | FDA Global Substance Registration System (GSRS) | |
URL | https://gsrs.ncats.nih.gov/ginas/app/beta/substances/6U704SC0N2 | |
Description | The FDA Global Substance Registration System (GSRS) enables the efficient and accurate exchange of information on what substances are in regulated products. Instead of relying on names, which vary across regulatory domains, countries, and regions, the GSRS knowledge base makes it possible for substances to be defined by standardized, scientific descriptions. | |
Explanation | Unless otherwise noted, the contents of the FDA website (www.fda.gov), both text and graphics, are not copyrighted. They are in the public domain and may be republished, reprinted and otherwise used freely by anyone without the need to obtain permission from FDA. Credit to the U.S. Food and Drug Administration as the source is appreciated but not required. | |
Retrosynthesis Analysis
AI-Powered Synthesis Planning: Our tool employs the Template_relevance Pistachio, Template_relevance Bkms_metabolic, Template_relevance Pistachio_ringbreaker, Template_relevance Reaxys, Template_relevance Reaxys_biocatalysis model, leveraging a vast database of chemical reactions to predict feasible synthetic routes.
One-Step Synthesis Focus: Specifically designed for one-step synthesis, it provides concise and direct routes for your target compounds, streamlining the synthesis process.
Accurate Predictions: Utilizing the extensive PISTACHIO, BKMS_METABOLIC, PISTACHIO_RINGBREAKER, REAXYS, REAXYS_BIOCATALYSIS database, our tool offers high-accuracy predictions, reflecting the latest in chemical research and data.
Strategy Settings
Precursor scoring | Relevance Heuristic |
---|---|
Min. plausibility | 0.01 |
Model | Template_relevance |
Template Set | Pistachio/Bkms_metabolic/Pistachio_ringbreaker/Reaxys/Reaxys_biocatalysis |
Top-N result to add to graph | 6 |
Feasible Synthetic Routes
Q1: What is the role of diethyl sulfate in the synthesis of nitroguanidine from urea?
A1: Diethyl sulfate acts as an ethylating agent in the synthesis of nitroguanidine from urea. While the provided abstracts do not describe the complete synthesis process, they suggest a two-step reaction.
Disclaimer and Information on In-Vitro Research Products
Please be aware that all articles and product information presented on BenchChem are intended solely for informational purposes. The products available for purchase on BenchChem are specifically designed for in-vitro studies, which are conducted outside of living organisms. In-vitro studies, derived from the Latin term "in glass," involve experiments performed in controlled laboratory settings using cells or tissues. It is important to note that these products are not categorized as medicines or drugs, and they have not received approval from the FDA for the prevention, treatment, or cure of any medical condition, ailment, or disease. We must emphasize that any form of bodily introduction of these products into humans or animals is strictly prohibited by law. It is essential to adhere to these guidelines to ensure compliance with legal and ethical standards in research and experimentation.