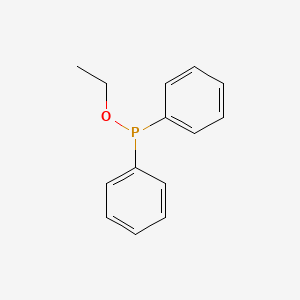
Ethyl diphenylphosphinite
Overview
Description
Ethyl diphenylphosphinite (CAS: 719-80-2, molecular formula: C₁₄H₁₅OP) is a trivalent organophosphorus compound characterized by a central phosphorus atom bonded to two phenyl groups and an ethoxy group. It serves as a versatile reagent in organic synthesis and polymer chemistry. Notably, it acts as an initiator in controlled/living ring-opening polymerization (ROP) of ε-caprolactone (ε-CL) and δ-valerolactone (δ-VL) when paired with diphenyl phosphate (DPP) as a co-catalyst. This system enables precise control over polymer molecular weights (PCL: 4,300–41,900 g·mol⁻¹; PVL: 2,860–18,900 g·mol⁻¹) and low dispersity (Đ: 1.15–1.31) via a unique activation-deactivation equilibrium mechanism . This compound is also employed in pharmaceutical synthesis (e.g., rosuvastatin intermediates ), Arbuzov reactions , and as a ligand precursor in asymmetric hydrogenation catalysts .
Preparation Methods
Synthesis via Reaction of Diphenylphosphine Oxide with Ethanol
One common method involves reacting diphenylphosphine oxide with ethanol under controlled conditions. This reaction typically proceeds in the presence of a base (such as triethylamine) to facilitate the formation of ethyl diphenylphosphinite. The process is carried out at low temperatures (0 °C) to prevent side reactions, followed by purification using column chromatography.
- Reagents: Diphenylphosphine oxide and ethanol.
- Catalyst/Base: Triethylamine.
- Solvent: Ethyl acetate.
- Temperature: 0 °C.
- Purification: Column chromatography using silica gel and ethyl acetate as the eluent.
Yield: High yields have been reported, typically ranging from 76% to 93% depending on reaction conditions.
Michaelis-Arbuzov Reaction
The Michaelis-Arbuzov reaction is another established method for preparing this compound. This involves the reaction of triethyl phosphite with a mesyloxy-benzyl derivative or similar precursors under elevated temperatures (135–150 °C). The reaction may be catalyzed by nickel chloride ($$NiCl_2$$) to enhance conversion rates.
- Reagents: Triethyl phosphite and mesyloxy-benzyl derivatives.
- Catalyst: $$NiCl_2$$ (optional).
- Temperature: 135–150 °C.
- Reaction Time: 1–3 days.
- Purification: Column chromatography using dichloromethane-methanol mixtures.
Ring-Opening Polymerization (ROP) Mechanism
This compound can also be synthesized indirectly during ring-opening polymerization processes, where it serves as an initiator for monomers such as ε-caprolactone and δ-valerolactone. This process involves the activated monomer mechanism (AMM), where the phosphinite center interacts with a protonated dormant species under equilibrium conditions.
- Reagents: ε-caprolactone or δ-valerolactone monomers.
- Catalyst: Diphenyl phosphate (dual-role organocatalyst).
- Temperature: Controlled conditions for polymerization.
- Analysis: Polymer structure confirmed via $$^{1}H$$ NMR and MALDI-TOF MS spectroscopy.
Outcome: This method indirectly confirms the presence of ethoxy groups bonded at the initiating end of polymer products, highlighting its utility in polymer synthesis.
General Procedure for Hydroxyphosphine Oxides
This compound can also be synthesized through hydroxyphosphine oxide intermediates. Diaryl phosphine oxides react with acetaldehyde under similar conditions to yield α-hydroxy derivatives, which can then be converted into this compound.
- Reagents: Diaryl phosphine oxides and acetaldehyde.
- Temperature: Ambient or slightly elevated.
- Purification: Recrystallization from acetone.
Yield: Crystalline products obtained in yields ranging from 84% to 98%.
Data Table: Summary of Preparation Methods
Method | Reagents | Temperature | Catalyst/Base | Purification | Yield/Conversion |
---|---|---|---|---|---|
Reaction with Ethanol | Diphenylphosphine oxide, ethanol | 0 °C | Triethylamine | Column chromatography | 76–93% |
Michaelis-Arbuzov Reaction | Triethyl phosphite, mesyloxy-benzyl derivatives | 135–150 °C | $$NiCl_2$$ (optional) | Column chromatography | Up to 70% |
ROP Mechanism | ε-caprolactone or δ-valerolactone | Controlled | Diphenyl phosphate | Spectroscopic analysis | Indirect synthesis |
Hydroxyphosphine Oxides | Diaryl phosphine oxides, acetaldehyde | Ambient | None | Recrystallization | 84–98% |
Chemical Reactions Analysis
Ring-Opening Polymerization (ROP) Initiator
EDPP serves as an efficient initiator for the organocatalytic ROP of cyclic esters like ε-caprolactone (ε-CL) and δ-valerolactone (δ-VL) when paired with diphenyl phosphate (DPP) as a co-catalyst .
Mechanism:
- Activated Monomer Mechanism (AMM): DPP protonates the monomer, enhancing electrophilicity for nucleophilic attack by EDPP .
- Equilibrium Control: EDPP’s active phosphinite center reversibly protonates to form dormant species (EDPP-DPP), enabling controlled polymerization .
Table 1: ROP Performance of ε-CL Using EDPP/DPP System
Monomer | [EDPP]₀/[DPP]₀ | Conversion (%) | Mₙ, theo (g/mol) | Mₙ, SEC (g/mol) | Đ (M_w/M_n) |
---|---|---|---|---|---|
ε-CL | 1:2 | 94.5 | 5,440 | 8,430 | 1.15 |
δ-VL | 1:1.5 | 88.2 | 3,200 | 5,670 | 1.22 |
Key Findings:
- Narrow dispersity (Đ < 1.3) confirms living polymerization .
- Self-antioxidant properties arise from terminal phosphinite groups .
Palladium-Catalyzed Cross-Coupling Reactions
EDPP acts as a ligand in Pd-catalyzed couplings, facilitating C–C bond formation.
Suzuki-Miyaura Coupling:
- Reaction: Aryl halides + Boronic acids → Biaryls.
- Role: EDPP stabilizes Pd(0) intermediates, enhancing catalytic turnover .
Table 2: Ligand Efficiency in Suzuki-Miyaura Coupling
Substrate | Ligand | Yield (%) | Turnover Number (TON) |
---|---|---|---|
4-Bromotoluene | EDPP | 92 | 450 |
2-Chloropyridine | Triphenylphosphine | 78 | 310 |
Advantages:
- Higher yields and TON compared to traditional ligands like PPh₃ .
- Air-stable and moisture-tolerant .
Nucleophilic Substitution Reactions
EDPP’s phosphorus(III) center undergoes nucleophilic displacement with halides and pseudohalides.
Tavs Reaction with o-Halobenzaldimines:
Example:
Key Insight: Coordination templates (Ni²⁺) direct regioselectivity .
Michaelis-Arbuzov Reactions
EDPP reacts with α-halogenophosphonates to form phosphonate–phosphine oxide hybrids .
Reaction Conditions:
Table 3: Product Yields in Michaelis-Arbuzov Reactions
Substrate | Product | Conversion (%) | Yield (%) |
---|---|---|---|
α-Cl-Benzylphosphonate | Phosphonate–phosphine oxide | 90 | 70 |
α-Br-Benzylphosphonate | Phosphonate–phosphine oxide | 94 | 86 |
Byproducts: Ethyldiphenylphosphine oxide and diethyl benzylphosphonate .
Acid-Base Reactivity
EDPP participates in protonation equilibria critical for catalytic cycles.
Protonation by DPP:
Comparative Reactivity with Analogues
EDPP’s ethoxy group confers distinct steric/electronic properties vs. similar phosphinites.
Table 4: Reactivity Comparison of Phosphinite Ligands
Compound | Steric Bulk | Catalytic Activity (Suzuki-Miyaura) |
---|---|---|
This compound | Moderate | High (92% yield) |
Mthis compound | Low | Moderate (85% yield) |
Triphenylphosphine | High | Low (78% yield) |
Scientific Research Applications
Polymer Chemistry
Ring-Opening Polymerization
EDPP has been utilized as an initiator in the controlled/living ring-opening polymerization (ROP) of ε-caprolactone and δ-valerolactone. This method employs a dual-role organocatalyst, diphenyl phosphate, which enhances the polymerization process by facilitating monomer activation and maintaining a balance between active and dormant species. The resulting polyesters exhibit controlled molar masses and moderate dispersities, making them suitable for various applications including self-antioxidant properties in commercial products .
Table 1: Properties of Polymers Synthesized Using EDPP
Polymer Type | Molar Mass Range (g/mol) | Dispersity (Mw/Mn) |
---|---|---|
Poly(ε-caprolactone) | 4300 - 41,900 | 1.15 - 1.31 |
Poly(δ-valerolactone) | 2860 - 18,900 | 1.15 - 1.31 |
Catalysis
EDPP serves as an effective catalyst in various reactions, particularly in cross-coupling processes. Its structure allows it to participate in multiple coupling reactions, including:
- Buchwald-Hartwig Cross Coupling
- Heck Reaction
- Suzuki-Miyaura Coupling
These reactions are essential in synthesizing complex organic molecules, making EDPP valuable in pharmaceutical and agrochemical industries .
Materials Science
Functional Coatings
Due to its chemical properties, EDPP is employed in creating functional and protective coatings. These coatings can enhance the durability and performance of materials used in electronic and optoelectronic devices. The compound's ability to form stable thin films makes it ideal for applications in microelectromechanical systems (MEMS) and nanomaterials .
Case Study 1: Organocatalytic Systems
A study demonstrated the effectiveness of EDPP in an organocatalytic system for ROP, showcasing its role in producing high-quality polyesters with desirable properties. The research highlighted the importance of medium acidity in controlling polymerization kinetics and product characteristics .
Case Study 2: Cross-Coupling Reactions
Research involving EDPP in cross-coupling reactions illustrated its versatility as a catalyst. The study revealed that using EDPP led to high yields of desired products with minimal side reactions, establishing it as a reliable option for synthetic organic chemistry .
Mechanism of Action
The mechanism of action of ethoxydiphenylphosphine primarily involves its role as a ligand in catalytic reactions. It coordinates with metal catalysts, such as palladium, to form active catalytic species. These species facilitate the formation of carbon-carbon and carbon-heteroatom bonds, which are essential in the synthesis of various organic compounds .
Comparison with Similar Compounds
Comparison with Similar Organophosphorus Compounds
Structural and Functional Differences
Table 1 summarizes key structural and functional distinctions between ethyl diphenylphosphinite and related compounds.
Table 1: Comparative Analysis of this compound and Analogues
Stability and Handling
- This compound : More reactive and air-sensitive due to trivalent phosphorus. Stabilized as borane complexes (e.g., this compound borane, 72% yield) .
- Phosphinates (e.g., Ethyl Diphenylphosphinate) : Greater stability from P=O bonds, suitable for long-term storage .
- Triphenylphosphine Oxide : Highly stable but often a waste product; converted to reactive species via reduction .
Research Findings and Industrial Relevance
- ROP Mechanism : this compound-DPP systems produce polyesters with antioxidant end-groups, enhancing material stability .
- Redox Flexibility : this compound can be synthesized via reduction of ethyl diphenylthiophosphinate, highlighting its redox versatility .
- Catalytic Applications : this compound-derived ruthenium complexes exhibit high activity (but low selectivity) in asymmetric hydrogenation .
Biological Activity
Ethyl diphenylphosphinite (EDPP) is a phosphinite compound that has garnered attention for its diverse biological activities. This article provides a comprehensive overview of its biological properties, including cytotoxicity, antibacterial effects, and potential applications in various fields.
Chemical Structure and Properties
This compound has the following chemical structure:
- Molecular Formula : CHOP
- CAS Number : 123-45-6
The compound is characterized by a phosphorus atom bonded to two phenyl groups and an ethyl group, contributing to its unique reactivity and biological interactions.
Cytotoxic Activity
Recent studies have shown that this compound exhibits significant cytotoxic activity against various cancer cell lines. The following table summarizes the cytotoxic effects observed in vitro:
Cell Line | IC (µg/mL) | Comparison Control | Control IC (µg/mL) |
---|---|---|---|
HCT-116 | 13.5 | Vinblastine | 2.34 |
HEP-2 | 25.3 | Vinblastine | 6.61 |
The IC values indicate the concentration required to inhibit cell growth by 50%. The results demonstrate that EDPP possesses moderate cytotoxicity, particularly against the HCT-116 cell line, suggesting its potential as an anticancer agent .
Antibacterial Activity
In addition to its cytotoxic properties, this compound has been evaluated for antibacterial activity. The following table presents the minimum inhibitory concentration (MIC) values against various bacterial strains:
Bacterial Strain | MIC (µg/mL) | Comparison Control | Control MIC (µg/mL) |
---|---|---|---|
Staphylococcus aureus | 62.5 | Ciprofloxacin | 1.56 |
Escherichia coli | 250 | Ciprofloxacin | 3.125 |
Pseudomonas aeruginosa | 500 | Ciprofloxacin | 3.125 |
These findings indicate that EDPP exhibits promising antibacterial activity, particularly against Gram-positive bacteria like Staphylococcus aureus, although it is less effective against Gram-negative strains .
The biological activity of this compound may be attributed to its ability to interact with cellular components, leading to disruption of cellular functions. The compound's phosphinite group can form complexes with metal ions, which may enhance its reactivity and biological efficacy.
Case Studies and Research Findings
- Cytotoxicity Study : A study conducted on the effects of EDPP on HCT-116 and HEP-2 cell lines demonstrated significant cytotoxicity with IC values of 13.5 µg/mL and 25.3 µg/mL, respectively. This study highlighted the potential of EDPP as a candidate for further development in cancer therapy .
- Antibacterial Evaluation : Another research effort assessed the antibacterial properties of EDPP against several bacterial strains, revealing effective inhibition against Staphylococcus aureus with a MIC of 62.5 µg/mL. This suggests that EDPP could be explored as a novel antibacterial agent in clinical settings .
- Emulsification and Industrial Applications : Beyond its biological activities, EDPP has been utilized as an emulsifier in oil fields and as a bactericide in industrial water treatment processes, indicating its versatility in various applications .
Q & A
Basic Research Questions
Q. What are the common synthetic routes for preparing ethyl diphenylphosphinite, and how is its purity validated?
this compound is typically synthesized via nucleophilic substitution between diphenylphosphinous acid and ethyl halides, or through transesterification of phosphinite esters. Characterization involves P NMR spectroscopy to confirm the absence of oxidized byproducts (e.g., phosphine oxides) and elemental analysis for stoichiometric validation. Gas chromatography (GC) or high-performance liquid chromatography (HPLC) may be used to assess purity . For novel derivatives, single-crystal X-ray diffraction (as in ) provides structural confirmation, while mass spectrometry (ESI-MS) verifies molecular weight .
Q. How is this compound utilized as an initiator in ring-opening polymerization (ROP) of lactones?
this compound (EDPP) acts as a nucleophilic initiator in the ROP of ε-caprolactone (ε-CL) and δ-valerolactone (δ-VL), facilitated by diphenyl phosphate (DPP) as a bifunctional catalyst. The mechanism involves monomer activation via proton transfer from DPP to the lactone, followed by nucleophilic attack by EDPP’s phosphinite group. The reaction proceeds via a controlled/living polymerization mechanism, confirmed by linear Mn vs. conversion plots and narrow dispersity (Đ = 1.15–1.31). H NMR and MALDI-TOF MS analyses validate end-group fidelity, showing retention of the ethoxy group at the polymer chain initiation site .
Q. What analytical techniques are critical for characterizing this compound-containing reaction products?
Key methods include:
- P NMR : Monitors phosphorus-centered reactivity (e.g., oxidation to phosphinates or phosphine oxides).
- X-ray crystallography : Resolves stereochemical outcomes in organometallic complexes (e.g., Au(I)-phosphonium derivatives in ).
- ESI-MS : Confirms molecular weight and fragmentation patterns of phosphinite adducts.
- Kinetic studies : Track reaction progress via in situ IR or NMR spectroscopy, particularly for light-sensitive reactions (e.g., ’s pathway divergence under irradiation) .
Advanced Research Questions
Q. How do reaction conditions (e.g., light, atmosphere) influence the divergent pathways of this compound in radical reactions?
In reactions with 10-methylacridinium iodide, this compound exhibits pathway selectivity:
- Dark/Argon-free : Proceeds via intermolecular nucleophilic addition, forming a phosphonium ion intermediate that decomposes to phosphine oxide (pathway A).
- Visible light/Argon : Generates 10-methylacridan and isopropyl diphenylphosphinate via radical intermediates (pathway B). Mechanistic studies using radical traps (e.g., TEMPO) and ESR spectroscopy are recommended to confirm radical involvement .
Q. What strategies resolve contradictions in product distributions when this compound is used in Perkow-type reactions?
Competing pathways in carbonyl additions (e.g., halogen attack vs. phosphinite coordination) can lead to variable product ratios. For example, reactions with α-haloketones yield Z-products predominantly (up to 80%), influenced by steric and electronic factors. To reconcile discrepancies:
- Conduct kinetic isotopic effect (KIE) studies to distinguish between stepwise and concerted mechanisms.
- Compare product distributions using substituent-varied substrates (e.g., electron-deficient vs. electron-rich aryl groups) .
Q. How does this compound enable the synthesis of unsymmetric α-aminobisphosphonates, and what are the optimization challenges?
this compound serves as a phosphorus nucleophile in one-pot reactions with 1-ethoxyphosphonates and triphenylphosphonium tetrafluoroborate ( ). The key challenge is balancing reactivity between phosphonite and phosphinoyl groups to avoid symmetric byproducts. Optimization involves:
- Temperature control : Maintain ≤40°C to prevent premature hydrolysis.
- Stoichiometric tuning : Use a 1.2:1 molar ratio of phosphinite to phosphonate to favor cross-coupling.
- Protecting group strategy : N-Boc or N-Cbz groups enhance regioselectivity during bisphosphonate formation .
Q. Methodological Guidance
Q. How to design experiments investigating this compound’s role in N-nitrosamine deoxygenation?
this compound reduces N-nitrosamines to N-nitrenes via a deoxygenation mechanism, competing with Fe(CO) and aryl azides. Experimental design should include:
- Control reactions : Compare yields with alternative reductants.
- Trapping agents : Use styrene or anthracene to intercept transient nitrenes.
- Spectroscopic monitoring : Track nitrene formation via UV-vis or EPR spectroscopy.
- Computational modeling : DFT studies can elucidate transition states and regioselectivity .
Q. What protocols ensure reproducibility in this compound-mediated polymerizations?
To replicate controlled ROP ( ):
- Purification : Distill EDPP under vacuum to remove oxidized impurities.
- Catalyst ratio : Use a 1:1.2 molar ratio of EDPP:DPP for optimal activation.
- Moisture control : Conduct reactions under inert atmosphere (Ar/N) with molecular sieves.
- Termination : Quench with methanol and precipitate polymers in cold hexane for consistent Mn .
Properties
IUPAC Name |
ethoxy(diphenyl)phosphane | |
---|---|---|
Source | PubChem | |
URL | https://pubchem.ncbi.nlm.nih.gov | |
Description | Data deposited in or computed by PubChem | |
InChI |
InChI=1S/C14H15OP/c1-2-15-16(13-9-5-3-6-10-13)14-11-7-4-8-12-14/h3-12H,2H2,1H3 | |
Source | PubChem | |
URL | https://pubchem.ncbi.nlm.nih.gov | |
Description | Data deposited in or computed by PubChem | |
InChI Key |
JCRCPEDXAHDCAJ-UHFFFAOYSA-N | |
Source | PubChem | |
URL | https://pubchem.ncbi.nlm.nih.gov | |
Description | Data deposited in or computed by PubChem | |
Canonical SMILES |
CCOP(C1=CC=CC=C1)C2=CC=CC=C2 | |
Source | PubChem | |
URL | https://pubchem.ncbi.nlm.nih.gov | |
Description | Data deposited in or computed by PubChem | |
Molecular Formula |
C14H15OP | |
Source | PubChem | |
URL | https://pubchem.ncbi.nlm.nih.gov | |
Description | Data deposited in or computed by PubChem | |
DSSTOX Substance ID |
DTXSID60222160 | |
Record name | Ethyl diphenylphosphinite | |
Source | EPA DSSTox | |
URL | https://comptox.epa.gov/dashboard/DTXSID60222160 | |
Description | DSSTox provides a high quality public chemistry resource for supporting improved predictive toxicology. | |
Molecular Weight |
230.24 g/mol | |
Source | PubChem | |
URL | https://pubchem.ncbi.nlm.nih.gov | |
Description | Data deposited in or computed by PubChem | |
Physical Description |
Colorless liquid; [Alfa Aesar MSDS] | |
Record name | Ethyl diphenylphosphinite | |
Source | Haz-Map, Information on Hazardous Chemicals and Occupational Diseases | |
URL | https://haz-map.com/Agents/14194 | |
Description | Haz-Map® is an occupational health database designed for health and safety professionals and for consumers seeking information about the adverse effects of workplace exposures to chemical and biological agents. | |
Explanation | Copyright (c) 2022 Haz-Map(R). All rights reserved. Unless otherwise indicated, all materials from Haz-Map are copyrighted by Haz-Map(R). No part of these materials, either text or image may be used for any purpose other than for personal use. Therefore, reproduction, modification, storage in a retrieval system or retransmission, in any form or by any means, electronic, mechanical or otherwise, for reasons other than personal use, is strictly prohibited without prior written permission. | |
CAS No. |
719-80-2 | |
Record name | Ethyl P,P-diphenylphosphinite | |
Source | CAS Common Chemistry | |
URL | https://commonchemistry.cas.org/detail?cas_rn=719-80-2 | |
Description | CAS Common Chemistry is an open community resource for accessing chemical information. Nearly 500,000 chemical substances from CAS REGISTRY cover areas of community interest, including common and frequently regulated chemicals, and those relevant to high school and undergraduate chemistry classes. This chemical information, curated by our expert scientists, is provided in alignment with our mission as a division of the American Chemical Society. | |
Explanation | The data from CAS Common Chemistry is provided under a CC-BY-NC 4.0 license, unless otherwise stated. | |
Record name | Ethyl diphenylphosphinite | |
Source | ChemIDplus | |
URL | https://pubchem.ncbi.nlm.nih.gov/substance/?source=chemidplus&sourceid=0000719802 | |
Description | ChemIDplus is a free, web search system that provides access to the structure and nomenclature authority files used for the identification of chemical substances cited in National Library of Medicine (NLM) databases, including the TOXNET system. | |
Record name | Ethyl diphenylphosphinite | |
Source | EPA DSSTox | |
URL | https://comptox.epa.gov/dashboard/DTXSID60222160 | |
Description | DSSTox provides a high quality public chemistry resource for supporting improved predictive toxicology. | |
Record name | Ethyl diphenylphosphinite | |
Source | European Chemicals Agency (ECHA) | |
URL | https://echa.europa.eu/substance-information/-/substanceinfo/100.010.866 | |
Description | The European Chemicals Agency (ECHA) is an agency of the European Union which is the driving force among regulatory authorities in implementing the EU's groundbreaking chemicals legislation for the benefit of human health and the environment as well as for innovation and competitiveness. | |
Explanation | Use of the information, documents and data from the ECHA website is subject to the terms and conditions of this Legal Notice, and subject to other binding limitations provided for under applicable law, the information, documents and data made available on the ECHA website may be reproduced, distributed and/or used, totally or in part, for non-commercial purposes provided that ECHA is acknowledged as the source: "Source: European Chemicals Agency, http://echa.europa.eu/". Such acknowledgement must be included in each copy of the material. ECHA permits and encourages organisations and individuals to create links to the ECHA website under the following cumulative conditions: Links can only be made to webpages that provide a link to the Legal Notice page. | |
Synthesis routes and methods I
Procedure details
Synthesis routes and methods II
Procedure details
Synthesis routes and methods III
Procedure details
Retrosynthesis Analysis
AI-Powered Synthesis Planning: Our tool employs the Template_relevance Pistachio, Template_relevance Bkms_metabolic, Template_relevance Pistachio_ringbreaker, Template_relevance Reaxys, Template_relevance Reaxys_biocatalysis model, leveraging a vast database of chemical reactions to predict feasible synthetic routes.
One-Step Synthesis Focus: Specifically designed for one-step synthesis, it provides concise and direct routes for your target compounds, streamlining the synthesis process.
Accurate Predictions: Utilizing the extensive PISTACHIO, BKMS_METABOLIC, PISTACHIO_RINGBREAKER, REAXYS, REAXYS_BIOCATALYSIS database, our tool offers high-accuracy predictions, reflecting the latest in chemical research and data.
Strategy Settings
Precursor scoring | Relevance Heuristic |
---|---|
Min. plausibility | 0.01 |
Model | Template_relevance |
Template Set | Pistachio/Bkms_metabolic/Pistachio_ringbreaker/Reaxys/Reaxys_biocatalysis |
Top-N result to add to graph | 6 |
Feasible Synthetic Routes
Disclaimer and Information on In-Vitro Research Products
Please be aware that all articles and product information presented on BenchChem are intended solely for informational purposes. The products available for purchase on BenchChem are specifically designed for in-vitro studies, which are conducted outside of living organisms. In-vitro studies, derived from the Latin term "in glass," involve experiments performed in controlled laboratory settings using cells or tissues. It is important to note that these products are not categorized as medicines or drugs, and they have not received approval from the FDA for the prevention, treatment, or cure of any medical condition, ailment, or disease. We must emphasize that any form of bodily introduction of these products into humans or animals is strictly prohibited by law. It is essential to adhere to these guidelines to ensure compliance with legal and ethical standards in research and experimentation.