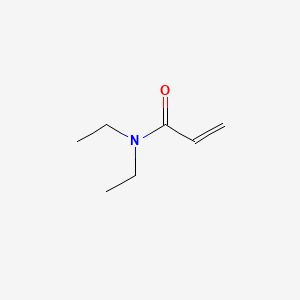
N,N-Diethylacrylamide
Overview
Description
N,N-Diethylacrylamide (DEAA) is an acrylamide monomer used to make polymers for drug delivery, thermal responsive polymers, and solutions with specifically tuned Lower Critical Solution Temperatures (LCSTs) . It has the empirical formula C7H13NO and a molecular weight of 127.18 .
Synthesis Analysis
DEAA can be synthesized through free radical polymerization . The process involves altering the concentration of N,N-diethylacrylamide (monomer), ammonium peroxodisulfate (initiator), N,N′-methylbisacrylamide (cross-linker), and N,N,N′,N′-tetramethylethylenediamine (accelerator) .Molecular Structure Analysis
The molecular structure of DEAA includes a carbonyl group (C=O) and an amide group (CONH2), which contribute to its reactivity and thermoresponsive behavior .Chemical Reactions Analysis
DEAA exhibits thermoresponsive behavior in aqueous solutions . The Lower Critical Solution Temperature (LCST) of the samples increases with increasing molecular weight and remains more or less constant above a critical molecular weight .Physical And Chemical Properties Analysis
DEAA is a liquid at room temperature with a density of 0.924 g/mL at 25 °C . It has a refractive index of 1.468 . The LCST of DEAA-based polymers can be tuned by altering the molecular weight and concentration .Scientific Research Applications
Thermoresponsive Polymers
N,N-Diethylacrylamide is used in the synthesis of thermoresponsive polymers . These polymers have the ability to change their properties in response to changes in temperature. For instance, Poly(N,N-diethylacrylamide-co-glycidyl methacrylate) (P(DEAAm-co-GMA)) is a thermoresponsive copolymer synthesized using N,N-Diethylacrylamide . This copolymer has been found to possess LCST-type thermoresponsive behavior with small extent of hysteresis .
Bioconjugation
The thermoresponsive copolymer P(DEAAm-co-GMA) has been used for bioconjugation with α-chymotrypsin, leading to enzyme–polymer nanoparticle (EPNP) with average size of 56.9 nm . This EPNP also shows reversible thermoresponsive behavior with somewhat higher critical solution temperature than that of the unreacted P(DEAAm-co-GMA) .
Enhanced Enzyme Stability
The bioconjugation of α-chymotrypsin with P(DEAAm-co-GMA) has been found to significantly enhance the pH and thermal stability of the enzyme .
Thermal Phase Transition Study
N,N-Diethylacrylamide is used in the study of thermal phase transition of polymers in aqueous solution . For instance, the phase transition of poly(N,N-diethylacrylamide-co-N-ethylacrylamide) random copolymers has been studied .
Biomedical Applications
N,N-Diethylacrylamide is used in the synthesis of polymers for biomedical applications . These polymers can act as on-off switches for modulated drug delivery and tissue engineering .
Synthesis of Thermal Responsive Polymers
N,N-Diethylacrylamide is used to make thermal responsive polymers . These polymers have the ability to change their properties in response to changes in temperature .
Solutions with Tuned LCSTs
N,N-Diethylacrylamide is used to make solutions with specifically tuned Lower Critical Solution Temperatures (LCSTs) . LCST is the temperature below which the solution phase separates into two distinct phases .
Drug Delivery Systems
The polymers synthesized using N,N-Diethylacrylamide can be used for drug delivery . These polymers can encapsulate drugs and release them in a controlled manner .
Mechanism of Action
Target of Action
N,N-Diethylacrylamide (DEA) is primarily used in the synthesis of poly(N,N-diethylacrylamide) (PDEA), a thermoresponsive polymer . The primary targets of DEA are therefore the components involved in the polymerization process, including the initiator, cross-linker, and accelerator .
Mode of Action
DEA interacts with these targets through free radical polymerization . In this process, DEA (the monomer) reacts with an initiator to form a radical, which then reacts with other monomers to form a polymer chain . The cross-linker and accelerator are used to control the polymerization rate and the physical properties of the resulting polymer .
Biochemical Pathways
The polymerization of DEA affects the physical properties of the resulting PDEA, including its swelling characteristics and temperature responsiveness . These properties can be manipulated by altering the concentration of DEA, the initiator, the cross-linker, and the synthesis temperature .
Pharmacokinetics
The pharmacokinetics of PDEA, the polymer derived from DEA, are largely determined by its physical properties. For example, the swelling characteristics of PDEA can affect its drug release behavior . In one study, a PDEA hydrogel was found to release the anticancer drug doxorubicin more efficiently at physiological temperature .
Result of Action
The polymerization of DEA results in the formation of PDEA, a thermoresponsive polymer with potential applications in drug delivery . For example, PDEA hydrogels have been used to improve the release of anticancer drugs .
Action Environment
The action of DEA and the properties of the resulting PDEA are influenced by various environmental factors. For example, the temperature can affect the swelling behavior of PDEA and its drug release efficiency . Other factors, such as the pH and the presence of certain ions, can also affect the properties of PDEA .
Safety and Hazards
Future Directions
properties
IUPAC Name |
N,N-diethylprop-2-enamide | |
---|---|---|
Source | PubChem | |
URL | https://pubchem.ncbi.nlm.nih.gov | |
Description | Data deposited in or computed by PubChem | |
InChI |
InChI=1S/C7H13NO/c1-4-7(9)8(5-2)6-3/h4H,1,5-6H2,2-3H3 | |
Source | PubChem | |
URL | https://pubchem.ncbi.nlm.nih.gov | |
Description | Data deposited in or computed by PubChem | |
InChI Key |
OVHHHVAVHBHXAK-UHFFFAOYSA-N | |
Source | PubChem | |
URL | https://pubchem.ncbi.nlm.nih.gov | |
Description | Data deposited in or computed by PubChem | |
Canonical SMILES |
CCN(CC)C(=O)C=C | |
Source | PubChem | |
URL | https://pubchem.ncbi.nlm.nih.gov | |
Description | Data deposited in or computed by PubChem | |
Molecular Formula |
C7H13NO | |
Source | PubChem | |
URL | https://pubchem.ncbi.nlm.nih.gov | |
Description | Data deposited in or computed by PubChem | |
Related CAS |
29933-92-4 | |
Record name | Poly(N,N-diethylacrylamide) | |
Source | CAS Common Chemistry | |
URL | https://commonchemistry.cas.org/detail?cas_rn=29933-92-4 | |
Description | CAS Common Chemistry is an open community resource for accessing chemical information. Nearly 500,000 chemical substances from CAS REGISTRY cover areas of community interest, including common and frequently regulated chemicals, and those relevant to high school and undergraduate chemistry classes. This chemical information, curated by our expert scientists, is provided in alignment with our mission as a division of the American Chemical Society. | |
Explanation | The data from CAS Common Chemistry is provided under a CC-BY-NC 4.0 license, unless otherwise stated. | |
DSSTOX Substance ID |
DTXSID10181258 | |
Record name | N,N-Diethylacrylamide | |
Source | EPA DSSTox | |
URL | https://comptox.epa.gov/dashboard/DTXSID10181258 | |
Description | DSSTox provides a high quality public chemistry resource for supporting improved predictive toxicology. | |
Molecular Weight |
127.18 g/mol | |
Source | PubChem | |
URL | https://pubchem.ncbi.nlm.nih.gov | |
Description | Data deposited in or computed by PubChem | |
Product Name |
N,N-Diethylacrylamide | |
CAS RN |
2675-94-7 | |
Record name | N,N-Diethylacrylamide | |
Source | CAS Common Chemistry | |
URL | https://commonchemistry.cas.org/detail?cas_rn=2675-94-7 | |
Description | CAS Common Chemistry is an open community resource for accessing chemical information. Nearly 500,000 chemical substances from CAS REGISTRY cover areas of community interest, including common and frequently regulated chemicals, and those relevant to high school and undergraduate chemistry classes. This chemical information, curated by our expert scientists, is provided in alignment with our mission as a division of the American Chemical Society. | |
Explanation | The data from CAS Common Chemistry is provided under a CC-BY-NC 4.0 license, unless otherwise stated. | |
Record name | N,N-Diethylacrylamide | |
Source | ChemIDplus | |
URL | https://pubchem.ncbi.nlm.nih.gov/substance/?source=chemidplus&sourceid=0002675947 | |
Description | ChemIDplus is a free, web search system that provides access to the structure and nomenclature authority files used for the identification of chemical substances cited in National Library of Medicine (NLM) databases, including the TOXNET system. | |
Record name | 2675-94-7 | |
Source | DTP/NCI | |
URL | https://dtp.cancer.gov/dtpstandard/servlet/dwindex?searchtype=NSC&outputformat=html&searchlist=20951 | |
Description | The NCI Development Therapeutics Program (DTP) provides services and resources to the academic and private-sector research communities worldwide to facilitate the discovery and development of new cancer therapeutic agents. | |
Explanation | Unless otherwise indicated, all text within NCI products is free of copyright and may be reused without our permission. Credit the National Cancer Institute as the source. | |
Record name | N,N-Diethylacrylamide | |
Source | EPA DSSTox | |
URL | https://comptox.epa.gov/dashboard/DTXSID10181258 | |
Description | DSSTox provides a high quality public chemistry resource for supporting improved predictive toxicology. | |
Record name | N,N-diethylacrylamide | |
Source | European Chemicals Agency (ECHA) | |
URL | https://echa.europa.eu/substance-information/-/substanceinfo/100.204.730 | |
Description | The European Chemicals Agency (ECHA) is an agency of the European Union which is the driving force among regulatory authorities in implementing the EU's groundbreaking chemicals legislation for the benefit of human health and the environment as well as for innovation and competitiveness. | |
Explanation | Use of the information, documents and data from the ECHA website is subject to the terms and conditions of this Legal Notice, and subject to other binding limitations provided for under applicable law, the information, documents and data made available on the ECHA website may be reproduced, distributed and/or used, totally or in part, for non-commercial purposes provided that ECHA is acknowledged as the source: "Source: European Chemicals Agency, http://echa.europa.eu/". Such acknowledgement must be included in each copy of the material. ECHA permits and encourages organisations and individuals to create links to the ECHA website under the following cumulative conditions: Links can only be made to webpages that provide a link to the Legal Notice page. | |
Record name | N,N-DIETHYLACRYLAMIDE | |
Source | FDA Global Substance Registration System (GSRS) | |
URL | https://gsrs.ncats.nih.gov/ginas/app/beta/substances/3552P2JF26 | |
Description | The FDA Global Substance Registration System (GSRS) enables the efficient and accurate exchange of information on what substances are in regulated products. Instead of relying on names, which vary across regulatory domains, countries, and regions, the GSRS knowledge base makes it possible for substances to be defined by standardized, scientific descriptions. | |
Explanation | Unless otherwise noted, the contents of the FDA website (www.fda.gov), both text and graphics, are not copyrighted. They are in the public domain and may be republished, reprinted and otherwise used freely by anyone without the need to obtain permission from FDA. Credit to the U.S. Food and Drug Administration as the source is appreciated but not required. | |
Synthesis routes and methods I
Procedure details
Synthesis routes and methods II
Procedure details
Retrosynthesis Analysis
AI-Powered Synthesis Planning: Our tool employs the Template_relevance Pistachio, Template_relevance Bkms_metabolic, Template_relevance Pistachio_ringbreaker, Template_relevance Reaxys, Template_relevance Reaxys_biocatalysis model, leveraging a vast database of chemical reactions to predict feasible synthetic routes.
One-Step Synthesis Focus: Specifically designed for one-step synthesis, it provides concise and direct routes for your target compounds, streamlining the synthesis process.
Accurate Predictions: Utilizing the extensive PISTACHIO, BKMS_METABOLIC, PISTACHIO_RINGBREAKER, REAXYS, REAXYS_BIOCATALYSIS database, our tool offers high-accuracy predictions, reflecting the latest in chemical research and data.
Strategy Settings
Precursor scoring | Relevance Heuristic |
---|---|
Min. plausibility | 0.01 |
Model | Template_relevance |
Template Set | Pistachio/Bkms_metabolic/Pistachio_ringbreaker/Reaxys/Reaxys_biocatalysis |
Top-N result to add to graph | 6 |
Feasible Synthetic Routes
Disclaimer and Information on In-Vitro Research Products
Please be aware that all articles and product information presented on BenchChem are intended solely for informational purposes. The products available for purchase on BenchChem are specifically designed for in-vitro studies, which are conducted outside of living organisms. In-vitro studies, derived from the Latin term "in glass," involve experiments performed in controlled laboratory settings using cells or tissues. It is important to note that these products are not categorized as medicines or drugs, and they have not received approval from the FDA for the prevention, treatment, or cure of any medical condition, ailment, or disease. We must emphasize that any form of bodily introduction of these products into humans or animals is strictly prohibited by law. It is essential to adhere to these guidelines to ensure compliance with legal and ethical standards in research and experimentation.