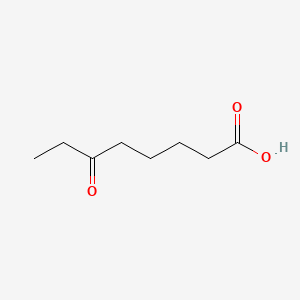
6-Oxo-octanoic acid
Overview
Description
6-Oxo-octanoic acid, also known as 6-keto-n-caprylic acid, is a chemical compound with the molecular formula C8H14O3 . It has an average mass of 158.195 Da and a mono-isotopic mass of 158.094299 Da .
Synthesis Analysis
The synthesis of compounds similar to 6-Oxo-octanoic acid has been described in various patents. For instance, the preparation of ethyl 6-oxo-8-chloro-caprylate involves an addition reaction on ethyl 6-chloro-6-oxo-caproate and ethylene in dichloroethane solution with aluminum trichloride as a catalyst . Another compound, SM-102, which is used in lipid nanoparticles for mRNA-based vaccines, is synthesized through an alkylation reaction where a secondary amine is combined with a lipid bromo ester .
Molecular Structure Analysis
The molecular structure of 6-Oxo-octanoic acid consists of 8 carbon atoms, 14 hydrogen atoms, and 3 oxygen atoms . It has several properties such as a density of 1.0±0.1 g/cm3, boiling point of 311.1±25.0 °C at 760 mmHg, and a flash point of 156.2±19.7 °C .
Physical And Chemical Properties Analysis
6-Oxo-octanoic acid has several physical and chemical properties. It has a density of 1.0±0.1 g/cm3, a boiling point of 311.1±25.0 °C at 760 mmHg, and a flash point of 156.2±19.7 °C . It also has a molar refractivity of 40.7±0.3 cm3, a polar surface area of 54 Å2, and a molar volume of 152.3±3.0 cm3 .
Scientific Research Applications
Pharmaceutical Excipients
6-Oxo-octanoic acid: is utilized in the synthesis of biodegradable cationic and ionizable cationic lipids. These lipids serve as auxiliary agents in pharmaceutical products, enhancing the delivery of therapeutic agents by improving cellular membrane permeability . The biodegradable nature of these lipids, when degraded to endogenous metabolites, minimizes their toxic potential, making them safer pharmaceutical excipients.
Antibacterial Agents
As a derivative of octanoic acid, 6-Oxo-octanoic acid may inherit antibacterial properties. Octanoic acid has been identified as an antibacterial agent effective against a range of bacteria . This suggests potential applications of 6-Oxo-octanoic acid in developing new antibacterial formulations.
Metabolite Research
6-Oxo-octanoic acid: can be a subject of study in metabolite research, particularly as a human metabolite. Understanding its role and metabolism in the human body can provide insights into various metabolic diseases and conditions .
Material Science
In material science, 6-Oxo-octanoic acid could be explored for the development of new materials with specific properties, such as biodegradability or amphiphilicity, which are desirable in a range of applications from packaging to medical devices .
Enzyme Function Studies
The compound’s interaction with enzymes, such as those involved in lipid metabolism, can be studied to understand the biochemical pathways and enzyme functions. This knowledge is crucial for developing drugs that target specific enzymes .
Spectroscopy and Structural Analysis
6-Oxo-octanoic acid: is a candidate for infrared spectroscopy studies to understand its structural properties and interactions with other molecules. Such research can lead to the development of new analytical techniques or materials .
Food Preservatives
Drawing from the applications of its parent compound, octanoic acid, 6-Oxo-octanoic acid could be investigated for its efficacy as a food preservative. Its potential biodegradability and safety profile make it a candidate for preserving food while minimizing health risks .
Cosmetic Industry
In the cosmetic industry, 6-Oxo-octanoic acid might be used in the formulation of skin care products due to its potential moisturizing properties and its role in lipid metabolism. Research into its effects on skin health could lead to innovative cosmetic products .
Safety and Hazards
Carboxylic acids, including 6-Oxo-octanoic acid, can cause severe skin burns and eye damage. They may also cause respiratory irritation . It is recommended to avoid breathing dust/fume/gas/mist/vapors/spray, to wash face, hands and any exposed skin thoroughly after handling, and to wear protective gloves/protective clothing/eye protection/face protection .
Mechanism of Action
Biochemical Pathways
6-Oxo-octanoic acid is likely involved in several biochemical pathways. For instance, keto acids appear in a wide variety of anabolic pathways in metabolism . In plants, 5-oxo-octanoic acid, a similar compound, is converted in enzymatic and non-enzymatic steps into the cyclic class of coniine alkaloids .
Pharmacokinetics
Similar compounds, such as sm-102, an ionizable cationic amino lipid, have been used in the formation of lipid nanoparticles for the delivery of mrna-based vaccines . The pharmacokinetics of such compounds often involve complex interactions with biological membranes, intracellular trafficking, and eventual metabolism and clearance .
Action Environment
The action of 6-Oxo-octanoic acid, like many biochemical compounds, can be influenced by various environmental factors. For instance, the pH, temperature, and presence of other molecules can affect the stability, efficacy, and action of the compound .
properties
IUPAC Name |
6-oxooctanoic acid | |
---|---|---|
Source | PubChem | |
URL | https://pubchem.ncbi.nlm.nih.gov | |
Description | Data deposited in or computed by PubChem | |
InChI |
InChI=1S/C8H14O3/c1-2-7(9)5-3-4-6-8(10)11/h2-6H2,1H3,(H,10,11) | |
Source | PubChem | |
URL | https://pubchem.ncbi.nlm.nih.gov | |
Description | Data deposited in or computed by PubChem | |
InChI Key |
PUOYTVHCFIDKJM-UHFFFAOYSA-N | |
Source | PubChem | |
URL | https://pubchem.ncbi.nlm.nih.gov | |
Description | Data deposited in or computed by PubChem | |
Canonical SMILES |
CCC(=O)CCCCC(=O)O | |
Source | PubChem | |
URL | https://pubchem.ncbi.nlm.nih.gov | |
Description | Data deposited in or computed by PubChem | |
Molecular Formula |
C8H14O3 | |
Source | PubChem | |
URL | https://pubchem.ncbi.nlm.nih.gov | |
Description | Data deposited in or computed by PubChem | |
DSSTOX Substance ID |
DTXSID60195143 | |
Record name | Octanoic acid, 6-oxo- | |
Source | EPA DSSTox | |
URL | https://comptox.epa.gov/dashboard/DTXSID60195143 | |
Description | DSSTox provides a high quality public chemistry resource for supporting improved predictive toxicology. | |
Molecular Weight |
158.19 g/mol | |
Source | PubChem | |
URL | https://pubchem.ncbi.nlm.nih.gov | |
Description | Data deposited in or computed by PubChem | |
Product Name |
6-Oxo-octanoic acid | |
CAS RN |
4233-57-2 | |
Record name | Octanoic acid, 6-oxo- | |
Source | ChemIDplus | |
URL | https://pubchem.ncbi.nlm.nih.gov/substance/?source=chemidplus&sourceid=0004233572 | |
Description | ChemIDplus is a free, web search system that provides access to the structure and nomenclature authority files used for the identification of chemical substances cited in National Library of Medicine (NLM) databases, including the TOXNET system. | |
Record name | Octanoic acid, 6-oxo- | |
Source | EPA DSSTox | |
URL | https://comptox.epa.gov/dashboard/DTXSID60195143 | |
Description | DSSTox provides a high quality public chemistry resource for supporting improved predictive toxicology. | |
Retrosynthesis Analysis
AI-Powered Synthesis Planning: Our tool employs the Template_relevance Pistachio, Template_relevance Bkms_metabolic, Template_relevance Pistachio_ringbreaker, Template_relevance Reaxys, Template_relevance Reaxys_biocatalysis model, leveraging a vast database of chemical reactions to predict feasible synthetic routes.
One-Step Synthesis Focus: Specifically designed for one-step synthesis, it provides concise and direct routes for your target compounds, streamlining the synthesis process.
Accurate Predictions: Utilizing the extensive PISTACHIO, BKMS_METABOLIC, PISTACHIO_RINGBREAKER, REAXYS, REAXYS_BIOCATALYSIS database, our tool offers high-accuracy predictions, reflecting the latest in chemical research and data.
Strategy Settings
Precursor scoring | Relevance Heuristic |
---|---|
Min. plausibility | 0.01 |
Model | Template_relevance |
Template Set | Pistachio/Bkms_metabolic/Pistachio_ringbreaker/Reaxys/Reaxys_biocatalysis |
Top-N result to add to graph | 6 |
Feasible Synthetic Routes
Q & A
Q1: How is 6-oxo-octanoic acid formed during microbial menthol metabolism?
A1: The research by Madyastha et al. [] identifies 6-oxo-octanoic acid as a key intermediate in the bacterial degradation of (-)-menthol. They propose a pathway where (-)-menthol is initially oxidized to menthone. Further degradation leads to the formation of 3,7-dimethyl-6-hydroxyoctanoic acid, which is subsequently oxidized to 3,7-dimethyl-6-oxo-octanoic acid. This compound is thus a product of a multi-step enzymatic oxidation process. Interestingly, this pathway also appears to be involved in the metabolism of menthane-3,4-diol, which is converted to 6-oxo-octanoic acid and 4-hydroxy-3-keto-p-menthane by the same bacterium [].
Disclaimer and Information on In-Vitro Research Products
Please be aware that all articles and product information presented on BenchChem are intended solely for informational purposes. The products available for purchase on BenchChem are specifically designed for in-vitro studies, which are conducted outside of living organisms. In-vitro studies, derived from the Latin term "in glass," involve experiments performed in controlled laboratory settings using cells or tissues. It is important to note that these products are not categorized as medicines or drugs, and they have not received approval from the FDA for the prevention, treatment, or cure of any medical condition, ailment, or disease. We must emphasize that any form of bodily introduction of these products into humans or animals is strictly prohibited by law. It is essential to adhere to these guidelines to ensure compliance with legal and ethical standards in research and experimentation.