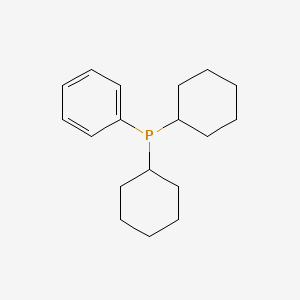
Dicyclohexylphenylphosphine
Overview
Description
Dicyclohexylphenylphosphine is a chemical compound with the linear formula (C6H11)2PC6H5 . It has a molecular weight of 274.38 . It is used as a pharmaceutical intermediate .
Molecular Structure Analysis
The molecular structure of Dicyclohexylphenylphosphine is represented by the formula (C6H11)2PC6H5 . This indicates that it consists of two cyclohexyl groups and one phenyl group attached to a phosphorus atom .Chemical Reactions Analysis
Dicyclohexylphenylphosphine is suitable for various types of coupling reactions, including Buchwald-Hartwig Cross Coupling, Heck Reaction, Hiyama Coupling, Negishi Coupling, Sonogashira Coupling, Stille Coupling, and Suzuki-Miyaura Coupling .Physical And Chemical Properties Analysis
Dicyclohexylphenylphosphine is a solid crystal at room temperature . It has a melting point range of 58-60°C . It is insoluble in water .Scientific Research Applications
Organic Synthesis
Dicyclohexylphenylphosphine: is a valuable ligand in organic synthesis, particularly in Palladium-catalyzed cross-coupling reactions . It facilitates various types of coupling reactions, including:
Pharmaceutical Intermediates
In the pharmaceutical industry, Dicyclohexylphenylphosphine serves as an intermediate in the synthesis of various drugs . Its role is pivotal in the development of new medicinal compounds, where it can help improve the efficacy and stability of the active pharmaceutical ingredients.
Safety and Hazards
Mechanism of Action
Target of Action
Dicyclohexylphenylphosphine is a phosphine ligand . Ligands are ions or molecules that bind to a central metal atom to form a coordination complex. The primary target of Dicyclohexylphenylphosphine is therefore the metal atom in these complexes.
Mode of Action
As a phosphine ligand, Dicyclohexylphenylphosphine binds to the metal atom in a coordination complex through its phosphorus atom . This binding can alter the electronic structure of the metal atom, influencing its reactivity and catalytic properties.
Biochemical Pathways
Dicyclohexylphenylphosphine is often used in various types of coupling reactions, including Buchwald-Hartwig, Heck, Hiyama, Negishi, Sonogashira, Stille, and Suzuki-Miyaura Couplings . These reactions are crucial in the synthesis of complex organic compounds, including pharmaceuticals and polymers.
Result of Action
The binding of Dicyclohexylphenylphosphine to a metal atom can significantly influence the outcome of a chemical reaction. By altering the electronic structure of the metal atom, it can enhance the atom’s ability to catalyze certain reactions, leading to the efficient formation of desired products .
Action Environment
The action of Dicyclohexylphenylphosphine can be influenced by various environmental factors. For example, the nature of the solvent can affect its solubility and therefore its availability to participate in reactions. Additionally, factors such as temperature and pH can influence the stability of the coordination complex and the rate of the reaction .
properties
IUPAC Name |
dicyclohexyl(phenyl)phosphane | |
---|---|---|
Source | PubChem | |
URL | https://pubchem.ncbi.nlm.nih.gov | |
Description | Data deposited in or computed by PubChem | |
InChI |
InChI=1S/C18H27P/c1-4-10-16(11-5-1)19(17-12-6-2-7-13-17)18-14-8-3-9-15-18/h1,4-5,10-11,17-18H,2-3,6-9,12-15H2 | |
Source | PubChem | |
URL | https://pubchem.ncbi.nlm.nih.gov | |
Description | Data deposited in or computed by PubChem | |
InChI Key |
VPLLTGLLUHLIHA-UHFFFAOYSA-N | |
Source | PubChem | |
URL | https://pubchem.ncbi.nlm.nih.gov | |
Description | Data deposited in or computed by PubChem | |
Canonical SMILES |
C1CCC(CC1)P(C2CCCCC2)C3=CC=CC=C3 | |
Source | PubChem | |
URL | https://pubchem.ncbi.nlm.nih.gov | |
Description | Data deposited in or computed by PubChem | |
Molecular Formula |
C18H27P | |
Source | PubChem | |
URL | https://pubchem.ncbi.nlm.nih.gov | |
Description | Data deposited in or computed by PubChem | |
DSSTOX Substance ID |
DTXSID0073372 | |
Record name | Phosphine, dicyclohexylphenyl- | |
Source | EPA DSSTox | |
URL | https://comptox.epa.gov/dashboard/DTXSID0073372 | |
Description | DSSTox provides a high quality public chemistry resource for supporting improved predictive toxicology. | |
Molecular Weight |
274.4 g/mol | |
Source | PubChem | |
URL | https://pubchem.ncbi.nlm.nih.gov | |
Description | Data deposited in or computed by PubChem | |
Product Name |
Dicyclohexylphenylphosphine | |
CAS RN |
6476-37-5 | |
Record name | Dicyclohexylphenylphosphine | |
Source | CAS Common Chemistry | |
URL | https://commonchemistry.cas.org/detail?cas_rn=6476-37-5 | |
Description | CAS Common Chemistry is an open community resource for accessing chemical information. Nearly 500,000 chemical substances from CAS REGISTRY cover areas of community interest, including common and frequently regulated chemicals, and those relevant to high school and undergraduate chemistry classes. This chemical information, curated by our expert scientists, is provided in alignment with our mission as a division of the American Chemical Society. | |
Explanation | The data from CAS Common Chemistry is provided under a CC-BY-NC 4.0 license, unless otherwise stated. | |
Record name | Dicyclohexylphenylphosphine | |
Source | ChemIDplus | |
URL | https://pubchem.ncbi.nlm.nih.gov/substance/?source=chemidplus&sourceid=0006476375 | |
Description | ChemIDplus is a free, web search system that provides access to the structure and nomenclature authority files used for the identification of chemical substances cited in National Library of Medicine (NLM) databases, including the TOXNET system. | |
Record name | Phosphine, dicyclohexylphenyl- | |
Source | EPA DSSTox | |
URL | https://comptox.epa.gov/dashboard/DTXSID0073372 | |
Description | DSSTox provides a high quality public chemistry resource for supporting improved predictive toxicology. | |
Record name | Dicyclohexylphenylphosphine | |
Source | European Chemicals Agency (ECHA) | |
URL | https://echa.europa.eu/substance-information/-/substanceinfo/100.026.668 | |
Description | The European Chemicals Agency (ECHA) is an agency of the European Union which is the driving force among regulatory authorities in implementing the EU's groundbreaking chemicals legislation for the benefit of human health and the environment as well as for innovation and competitiveness. | |
Explanation | Use of the information, documents and data from the ECHA website is subject to the terms and conditions of this Legal Notice, and subject to other binding limitations provided for under applicable law, the information, documents and data made available on the ECHA website may be reproduced, distributed and/or used, totally or in part, for non-commercial purposes provided that ECHA is acknowledged as the source: "Source: European Chemicals Agency, http://echa.europa.eu/". Such acknowledgement must be included in each copy of the material. ECHA permits and encourages organisations and individuals to create links to the ECHA website under the following cumulative conditions: Links can only be made to webpages that provide a link to the Legal Notice page. | |
Retrosynthesis Analysis
AI-Powered Synthesis Planning: Our tool employs the Template_relevance Pistachio, Template_relevance Bkms_metabolic, Template_relevance Pistachio_ringbreaker, Template_relevance Reaxys, Template_relevance Reaxys_biocatalysis model, leveraging a vast database of chemical reactions to predict feasible synthetic routes.
One-Step Synthesis Focus: Specifically designed for one-step synthesis, it provides concise and direct routes for your target compounds, streamlining the synthesis process.
Accurate Predictions: Utilizing the extensive PISTACHIO, BKMS_METABOLIC, PISTACHIO_RINGBREAKER, REAXYS, REAXYS_BIOCATALYSIS database, our tool offers high-accuracy predictions, reflecting the latest in chemical research and data.
Strategy Settings
Precursor scoring | Relevance Heuristic |
---|---|
Min. plausibility | 0.01 |
Model | Template_relevance |
Template Set | Pistachio/Bkms_metabolic/Pistachio_ringbreaker/Reaxys/Reaxys_biocatalysis |
Top-N result to add to graph | 6 |
Feasible Synthetic Routes
Disclaimer and Information on In-Vitro Research Products
Please be aware that all articles and product information presented on BenchChem are intended solely for informational purposes. The products available for purchase on BenchChem are specifically designed for in-vitro studies, which are conducted outside of living organisms. In-vitro studies, derived from the Latin term "in glass," involve experiments performed in controlled laboratory settings using cells or tissues. It is important to note that these products are not categorized as medicines or drugs, and they have not received approval from the FDA for the prevention, treatment, or cure of any medical condition, ailment, or disease. We must emphasize that any form of bodily introduction of these products into humans or animals is strictly prohibited by law. It is essential to adhere to these guidelines to ensure compliance with legal and ethical standards in research and experimentation.