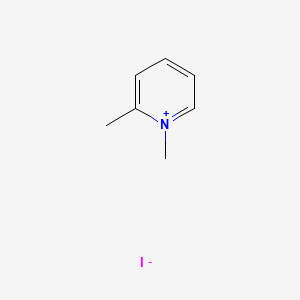
1,2-Dimethylpyridinium iodide
Overview
Description
Preparation Methods
1,2-Dimethylpyridinium iodide can be synthesized through the reaction of 1,2-dimethylpyridine with hydroiodic acid under alkaline conditions . The reaction typically involves heating the mixture to facilitate the formation of the iodide salt. Industrial production methods may involve similar synthetic routes but on a larger scale, ensuring the purity and yield of the final product .
Chemical Reactions Analysis
1,2-Dimethylpyridinium iodide undergoes various chemical reactions, including:
Demethylation and Recyclization: When heated with aqueous solutions of cyclic amines such as morpholine, piperidine, and piperazine, it undergoes demethylation and recyclization.
Substitution Reactions: It can participate in substitution reactions where the iodide ion is replaced by other nucleophiles under appropriate conditions.
Common reagents used in these reactions include sulfites of cyclic amines and other nucleophiles . The major products formed depend on the specific reaction conditions and reagents used.
Scientific Research Applications
1,2-Dimethylpyridinium iodide has several scientific research applications:
Catalysis: It is used as a catalyst in organic synthesis reactions.
Material Science:
Chemical Transformations: It is studied for its transformations under various conditions, providing insights into reaction mechanisms and pathways.
Mechanism of Action
The mechanism of action of 1,2-Dimethylpyridinium iodide involves its ability to undergo demethylation and recyclization in the presence of cyclic amines . This process can lead to the formation of various products depending on the reaction conditions. The molecular targets and pathways involved include the interaction with nucleophiles and the subsequent chemical transformations .
Comparison with Similar Compounds
1,2-Dimethylpyridinium iodide can be compared with other similar compounds such as:
3-Bromo-1-Ethylpyridinium Iodide: This compound also forms complexes with antimony and has similar applications in material science.
1,4-Dimethylpyridinium Iodide: This compound is used in the synthesis of fluorescent probes for imaging in live cells.
The uniqueness of this compound lies in its specific reactivity and applications in catalysis and material science .
Properties
IUPAC Name |
1,2-dimethylpyridin-1-ium;iodide | |
---|---|---|
Source | PubChem | |
URL | https://pubchem.ncbi.nlm.nih.gov | |
Description | Data deposited in or computed by PubChem | |
InChI |
InChI=1S/C7H10N.HI/c1-7-5-3-4-6-8(7)2;/h3-6H,1-2H3;1H/q+1;/p-1 | |
Source | PubChem | |
URL | https://pubchem.ncbi.nlm.nih.gov | |
Description | Data deposited in or computed by PubChem | |
InChI Key |
HNULCUYNXDDQCB-UHFFFAOYSA-M | |
Source | PubChem | |
URL | https://pubchem.ncbi.nlm.nih.gov | |
Description | Data deposited in or computed by PubChem | |
Canonical SMILES |
CC1=CC=CC=[N+]1C.[I-] | |
Source | PubChem | |
URL | https://pubchem.ncbi.nlm.nih.gov | |
Description | Data deposited in or computed by PubChem | |
Molecular Formula |
C7H10IN | |
Source | PubChem | |
URL | https://pubchem.ncbi.nlm.nih.gov | |
Description | Data deposited in or computed by PubChem | |
DSSTOX Substance ID |
DTXSID601007401 | |
Record name | 1,2-Dimethylpyridin-1-ium iodide | |
Source | EPA DSSTox | |
URL | https://comptox.epa.gov/dashboard/DTXSID601007401 | |
Description | DSSTox provides a high quality public chemistry resource for supporting improved predictive toxicology. | |
Molecular Weight |
235.07 g/mol | |
Source | PubChem | |
URL | https://pubchem.ncbi.nlm.nih.gov | |
Description | Data deposited in or computed by PubChem | |
CAS No. |
872-73-1 | |
Record name | Pyridinium, 1,2-dimethyl-, iodide (1:1) | |
Source | CAS Common Chemistry | |
URL | https://commonchemistry.cas.org/detail?cas_rn=872-73-1 | |
Description | CAS Common Chemistry is an open community resource for accessing chemical information. Nearly 500,000 chemical substances from CAS REGISTRY cover areas of community interest, including common and frequently regulated chemicals, and those relevant to high school and undergraduate chemistry classes. This chemical information, curated by our expert scientists, is provided in alignment with our mission as a division of the American Chemical Society. | |
Explanation | The data from CAS Common Chemistry is provided under a CC-BY-NC 4.0 license, unless otherwise stated. | |
Record name | 2-Picolinium, 1-methyl-, iodide | |
Source | ChemIDplus | |
URL | https://pubchem.ncbi.nlm.nih.gov/substance/?source=chemidplus&sourceid=0000872731 | |
Description | ChemIDplus is a free, web search system that provides access to the structure and nomenclature authority files used for the identification of chemical substances cited in National Library of Medicine (NLM) databases, including the TOXNET system. | |
Record name | 1,2-Dimethylpyridinium iodide | |
Source | DTP/NCI | |
URL | https://dtp.cancer.gov/dtpstandard/servlet/dwindex?searchtype=NSC&outputformat=html&searchlist=10494 | |
Description | The NCI Development Therapeutics Program (DTP) provides services and resources to the academic and private-sector research communities worldwide to facilitate the discovery and development of new cancer therapeutic agents. | |
Explanation | Unless otherwise indicated, all text within NCI products is free of copyright and may be reused without our permission. Credit the National Cancer Institute as the source. | |
Record name | 1,2-Dimethylpyridin-1-ium iodide | |
Source | EPA DSSTox | |
URL | https://comptox.epa.gov/dashboard/DTXSID601007401 | |
Description | DSSTox provides a high quality public chemistry resource for supporting improved predictive toxicology. | |
Retrosynthesis Analysis
AI-Powered Synthesis Planning: Our tool employs the Template_relevance Pistachio, Template_relevance Bkms_metabolic, Template_relevance Pistachio_ringbreaker, Template_relevance Reaxys, Template_relevance Reaxys_biocatalysis model, leveraging a vast database of chemical reactions to predict feasible synthetic routes.
One-Step Synthesis Focus: Specifically designed for one-step synthesis, it provides concise and direct routes for your target compounds, streamlining the synthesis process.
Accurate Predictions: Utilizing the extensive PISTACHIO, BKMS_METABOLIC, PISTACHIO_RINGBREAKER, REAXYS, REAXYS_BIOCATALYSIS database, our tool offers high-accuracy predictions, reflecting the latest in chemical research and data.
Strategy Settings
Precursor scoring | Relevance Heuristic |
---|---|
Min. plausibility | 0.01 |
Model | Template_relevance |
Template Set | Pistachio/Bkms_metabolic/Pistachio_ringbreaker/Reaxys/Reaxys_biocatalysis |
Top-N result to add to graph | 6 |
Feasible Synthetic Routes
Q1: What can you tell us about the structure of 3-hydroxy-4-hydroxyiminomethyl-5-hydroxymethyl-1,2-dimethylpyridinium iodide based on computational studies?
A: Computational studies using Density Functional Theory (DFT) with the B3LYP functional and LanL2DZ basis set were conducted on this compound [, , ]. These studies provided insights into its optimized geometry, vibrational frequencies, and frontier molecular orbitals (HOMO and LUMO). The results contribute to understanding its reactivity and potential interactions with other molecules.
Q2: How do the calculated electronic properties of 3-hydroxy-4-hydroxyiminomethyl-5-hydroxymethyl-1,2-dimethylpyridinium iodide relate to its potential reactivity?
A: The HOMO-LUMO energy gap, dipole moment, electronegativity, and chemical hardness and softness were calculated [, , ]. These electronic properties offer insights into the molecule's reactivity, stability, and potential for interactions with other chemical species. For example, the HOMO-LUMO gap is related to its kinetic stability and chemical reactivity.
Disclaimer and Information on In-Vitro Research Products
Please be aware that all articles and product information presented on BenchChem are intended solely for informational purposes. The products available for purchase on BenchChem are specifically designed for in-vitro studies, which are conducted outside of living organisms. In-vitro studies, derived from the Latin term "in glass," involve experiments performed in controlled laboratory settings using cells or tissues. It is important to note that these products are not categorized as medicines or drugs, and they have not received approval from the FDA for the prevention, treatment, or cure of any medical condition, ailment, or disease. We must emphasize that any form of bodily introduction of these products into humans or animals is strictly prohibited by law. It is essential to adhere to these guidelines to ensure compliance with legal and ethical standards in research and experimentation.