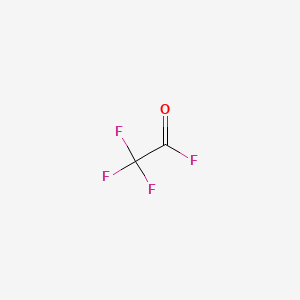
Trifluoroacetyl fluoride
Overview
Description
Trifluoroacetyl fluoride (TFAF, CAS 354-34-7) is a fluorinated acyl halide with the molecular formula C₂F₄O and a molecular weight of 116.01 g/mol . It is a volatile, reactive compound primarily used as an intermediate in organic synthesis and industrial processes, including pharmaceuticals, agrochemicals, and refrigerant blends . TFAF is produced via the atmospheric degradation of hydrofluorocarbons (HFCs) such as (E/Z)-1,2,3,3,3-pentafluoropropene (PFP), where it forms alongside formyl fluoride in reactions with chlorine (Cl) atoms . Its hydrolysis yields trifluoroacetic acid (TFA), a persistent environmental pollutant .
Preparation Methods
Gas-Phase Reactions
Method 1: Reaction with Trichloroacetyl Chloride
One prominent method involves the reaction of trichloroacetyl chloride with anhydrous hydrogen fluoride in the gas phase, typically using a chromium-containing catalyst. The process is characterized by:
Catalyst Composition : Chromium (III) hydroxide precipitated with magnesium hydroxide or magnesium oxide.
-
- Temperature: 200° to 400°C (preferably 250° to 370°C).
- Pressure: Atmospheric to slight superatmospheric (1 to 12 bar).
- Molar Ratio of Hydrogen Fluoride to Trichloroacetyl Chloride: Generally between 4:1 and 15:1.
-
- The reactants are passed through a preheater into a catalyst-packed reactor.
- The residence time in the reactor is typically between 1 to 60 seconds.
- The reaction yields trifluoroacetyl fluoride along with by-products like HCl and HF, which are subsequently condensed and purified through distillation.
Method 2: Continuous Preparation Method
A more recent development involves a continuous gas-phase preparation method that utilizes a high-activity catalyst supported on active carbon:
-
- The trifluoroethyl compound is gasified and mixed with anhydrous hydrogen fluoride before being introduced into a packed column containing the catalyst.
- The reaction occurs at temperatures ranging from 30°C to 100°C, allowing for efficient conversion.
Purification : The product is cooled and rectified to achieve a purity greater than 99.9%.
Liquid-Phase Reactions
Method: Hexafluorothioacetone Dimer Reaction
Another effective method for synthesizing this compound involves the liquid-phase reaction of hexafluorothioacetone dimer:
-
- Conducted in an aprotic solvent such as dimethylformamide.
- Requires a catalytic amount of alkali metal fluoride (e.g., potassium fluoride) and an oxidizing agent (e.g., silver oxide).
-
- Temperature: Typically maintained between 110°C and 140°C.
Outcome : The desired product is collected via distillation, often yielding high purity levels with minimal side products.
The following table summarizes key aspects of the different preparation methods for this compound:
Method | Phase | Key Reactants | Temperature Range | Catalyst Type | Purity Achieved |
---|---|---|---|---|---|
Gas-phase with Trichloroacetyl Chloride | Gas | Trichloroacetyl chloride, Hydrogen fluoride | 200° - 400°C | Chromium-containing | High |
Continuous Gas-phase Method | Gas | Trifluoroethyl compound, Hydrogen fluoride | 30° - 100°C | Active carbon-supported | >99.9% |
Liquid-phase with Hexafluorothioacetone Dimer | Liquid | Hexafluorothioacetone dimer, Alkali metal fluoride | 110° - 140°C | Alkali metal fluoride | High |
Chemical Reactions Analysis
Types of Reactions: Trifluoroacetyl fluoride undergoes various chemical reactions, including:
- Substitution Reactions: It reacts with nucleophiles such as alcohols and amines to form esters and amides .
CF3COF+R−OH→CF3COOR+HF
CF3COF+R−NH2→CF3CONHR+HF
Addition Reactions: It can add to alkenes and alkynes to form fluorinated compounds.
Decomposition Reactions: Thermal decomposition of this compound can produce carbonyl fluoride and other fluorinated products.
Common Reagents and Conditions:
Fluorinating Agents: Anhydrous hydrogen fluoride is commonly used in the synthesis of this compound.
Catalysts: Chromium-containing catalysts are used in industrial production methods.
Major Products Formed:
Esters and Amides: Formed through substitution reactions with alcohols and amines.
Fluorinated Compounds: Formed through addition reactions with alkenes and alkynes.
Scientific Research Applications
Trifluoroacetyl fluoride has several applications in scientific research:
Mechanism of Action
The mechanism of action of trifluoroacetyl fluoride involves its high reactivity with nucleophiles. The compound’s electrophilic carbonyl carbon is highly susceptible to nucleophilic attack, leading to the formation of various products . The presence of fluorine atoms enhances its reactivity and stability, making it a valuable intermediate in chemical reactions.
Comparison with Similar Compounds
Structural and Physical Properties
Table 1: Key Physical Properties of TFAF and Related Compounds
Key Observations :
- TFAF has a lower molecular weight and boiling point compared to TFAC due to fluorine's smaller atomic size and weaker intermolecular forces versus chlorine .
- TFA (a hydrolysis product of TFAF) is a liquid at room temperature, while TFAF and TFAC are gases, reflecting differences in polarity and hydrogen bonding .
Key Observations :
- Both TFAF and TFAC react with triethylamine to form β-diethylamino enones, but TFAC requires stoichiometric base (e.g., 2,6-lutidine) for efficient derivatization of amines and phenols .
- Hydrolysis products differ: TFAF releases HF (highly toxic), while TFAC releases HCl (less toxic but corrosive) .
Table 3: Hazard Profiles
Key Observations :
Biological Activity
Trifluoroacetyl fluoride (TFAF), a fluorinated organic compound with the formula , has garnered attention due to its potential biological activity and environmental implications. This article synthesizes available research findings regarding its biological effects, toxicity, and metabolic pathways.
This compound is primarily synthesized through the reaction of trichloroacetyl chloride with anhydrous hydrogen fluoride. This process yields TFAF with a high conversion rate and minimal decomposition of reactants . The compound is known to decompose in the atmosphere, leading to the formation of trifluoroacetic acid (TFA), which is a significant environmental pollutant .
In Vivo Studies
Research indicates that TFAF can influence metabolic processes in mammals. In studies involving rats, administration of TFA at doses of 150 mg/kg/day for 5-6 days resulted in notable changes in liver enzyme activities, including:
- Decreased hepatic glycogen content : 24% reduction.
- Altered enzyme activities :
- Pyruvate kinase decreased by 42%.
- Glycerol 1-phosphate oxidase increased by 125%.
These alterations suggest a significant impact on hepatic metabolism and energy storage . Furthermore, at higher doses (2000 mg/kg), TFAF was associated with decreased levels of hepatic NADPH and reduced glutathione, although these levels normalized after 24 hours .
In Vitro Studies
In vitro studies have demonstrated that TFAF affects protein binding in human serum albumin. At concentrations of 4 mM and 10 mM, it reduced the binding capacity for warfarin and phenytoin, suggesting potential implications for drug metabolism and efficacy . Additionally, cultured Morris rat hepatoma cells exposed to TFAF showed no significant impact on DNA or RNA synthesis at lower concentrations, indicating a selective effect on metabolic pathways rather than direct cytotoxicity .
Environmental Impact
This compound is persistent in the environment, often detected in water bodies and precipitation. Its degradation products, particularly trifluoroacetic acid, are prevalent due to atmospheric reactions involving hydrofluorocarbons (HFOs) . Studies have shown that TFA accumulates significantly in higher plants, particularly conifers, raising concerns about long-term ecological effects .
Case Study 1: Hepatic Effects in Rats
A study administered TFA at varying doses to rats over several days. The findings highlighted significant hepatic changes, including alterations in enzyme activities that could indicate potential hepatotoxicity or metabolic disruption.
Parameter | Control | TFA (150 mg/kg) | TFA (2000 mg/kg) |
---|---|---|---|
Hepatic Glycogen Content | Baseline | -24% | Not assessed |
Pyruvate Kinase Activity | Baseline | -42% | Not assessed |
Glycerol 1-Phosphate Oxidase | Baseline | +125% | Not assessed |
Hepatic NADPH Levels | Baseline | Not assessed | Decreased |
Reduced Glutathione Levels | Baseline | Not assessed | Decreased |
Case Study 2: Environmental Accumulation
Investigations into TFA levels in precipitation revealed concentrations ranging from 10 to 200 ng/L across various regions in Germany. This accumulation underscores the need for further studies on the ecological impacts of prolonged exposure to this compound in terrestrial and aquatic systems .
Q & A
Q. Basic: What are the primary synthetic routes for trifluoroacetyl fluoride, and how do reaction conditions influence yield and purity?
This compound (CF₃COF) is commonly synthesized via two main pathways:
- Trifluoroacetyl chloride fluorination : Reacting trifluoroacetyl chloride (CF₃COCl) with hydrogen fluoride (HF) under controlled conditions, typically in the presence of a chromium-based catalyst at 100–350°C .
- Electrochemical fluorination : Using the Simons method, acetyl chloride (CH₃COCl) is fluorinated with anhydrous HF, followed by hydrolysis of intermediate products to yield CF₃COF .
Yield optimization requires precise temperature control, catalyst activity monitoring, and purification steps (e.g., fractional distillation). Impurities like residual HCl or unreacted precursors can be minimized using gas-phase reactions and inert atmospheres .
Q. Basic: What spectroscopic and analytical techniques are most effective for characterizing this compound?
Key methods include:
- FTIR and Raman spectroscopy : To identify vibrational modes of C=O (≈1870 cm⁻¹) and C-F (≈1200–1300 cm⁻¹) bonds .
- NMR spectroscopy : ¹⁹F NMR resolves trifluoromethyl (-CF₃) and fluoride (-F) groups, with chemical shifts around -70 ppm and -140 ppm, respectively .
- Mass spectrometry (EI-MS) : Molecular ion peaks at m/z 116 (M⁺) and fragmentation patterns (e.g., loss of COF⁺) confirm structural integrity .
- Gas chromatography (GC) : Quantifies purity and detects volatile byproducts .
Q. Advanced: How can researchers design experiments to quantify this compound’s mass-transfer kinetics at air/water interfaces?
Methodological considerations include:
- Flow-tube reactors : Measure uptake coefficients (γ) by exposing CF₃COF to water surfaces under varying humidity and temperature. Use laser-induced fluorescence (LIF) or mass spectrometry for real-time detection .
- Langmuir trough systems : Monitor interfacial tension changes to infer adsorption dynamics. Pair with attenuated total reflectance (ATR)-FTIR for molecular-level insights .
- Computational modeling : Couple experimental data with density functional theory (DFT) to predict activation energies for hydrolysis or interfacial reactions .
Q. Advanced: What are the atmospheric degradation pathways of this compound, and how do UV photolysis experiments validate these mechanisms?
CF₃COF degrades via:
- Hydrolysis : Reacts with atmospheric moisture to form trifluoroacetic acid (TFA), a persistent environmental pollutant .
- Photolysis : Under UV-C (200–280 nm), CF₃COF undergoes C-F bond cleavage, producing CF₃ radicals and COF₂. Recent studies show secondary reactions can generate CF₄ (a potent greenhouse gas) .
Experimental validation involves:
Q. Advanced: What analytical challenges arise in detecting this compound in environmental samples, and how can they be mitigated?
Challenges include:
- Low ambient concentrations : Requires pre-concentration via cryogenic trapping or solid-phase microextraction (SPME) .
- Reactivity with solvents : Avoid aqueous matrices; use inert carriers like perfluorinated oils.
- Interference from fluorinated analogs : Differentiate using high-resolution mass spectrometry (HRMS) or tandem MS/MS .
Standardized protocols from agencies like ATSDR/NTP recommend isotope dilution (e.g., ¹³C-labeled CF₃COF) for quantification .
Q. Advanced: How can contradictory hydrolysis rate constants for this compound (e.g., Swain vs. Bunton) be resolved?
Discrepancies arise from:
- Solvent effects : Swain (1953) used non-polar solvents, reporting slower hydrolysis (k ≈ 10⁻⁴ s⁻¹), while Bunton (1966) observed faster rates (k ≈ 10⁻² s⁻¹) in polar media .
- Temperature dependence : Re-evaluate Arrhenius parameters using modern calorimetry.
- pH control : Hydrolysis accelerates under alkaline conditions due to nucleophilic attack by OH⁻. Use buffered solutions (pH 7–12) to standardize measurements .
Q. Advanced: What computational approaches are suitable for modeling this compound’s reaction mechanisms in catalytic systems?
Recommended strategies:
- DFT calculations : Simulate transition states for fluorination/hydrolysis pathways. Basis sets like 6-311++G(d,p) accurately model fluorine’s electronegativity .
- Molecular dynamics (MD) : Track diffusion coefficients in solvent-catalyst systems (e.g., Cr₂O₃ surfaces) .
- Kinetic Monte Carlo (kMC) : Predict yield trends under varying pressures and temperatures. Validate with experimental turnover frequencies (TOFs) .
Properties
IUPAC Name |
2,2,2-trifluoroacetyl fluoride | |
---|---|---|
Source | PubChem | |
URL | https://pubchem.ncbi.nlm.nih.gov | |
Description | Data deposited in or computed by PubChem | |
InChI |
InChI=1S/C2F4O/c3-1(7)2(4,5)6 | |
Source | PubChem | |
URL | https://pubchem.ncbi.nlm.nih.gov | |
Description | Data deposited in or computed by PubChem | |
InChI Key |
DCEPGADSNJKOJK-UHFFFAOYSA-N | |
Source | PubChem | |
URL | https://pubchem.ncbi.nlm.nih.gov | |
Description | Data deposited in or computed by PubChem | |
Canonical SMILES |
C(=O)(C(F)(F)F)F | |
Source | PubChem | |
URL | https://pubchem.ncbi.nlm.nih.gov | |
Description | Data deposited in or computed by PubChem | |
Molecular Formula |
C2F4O | |
Source | PubChem | |
URL | https://pubchem.ncbi.nlm.nih.gov | |
Description | Data deposited in or computed by PubChem | |
DSSTOX Substance ID |
DTXSID6059867 | |
Record name | Perfluoroacetyl fluoride | |
Source | EPA DSSTox | |
URL | https://comptox.epa.gov/dashboard/DTXSID6059867 | |
Description | DSSTox provides a high quality public chemistry resource for supporting improved predictive toxicology. | |
Molecular Weight |
116.01 g/mol | |
Source | PubChem | |
URL | https://pubchem.ncbi.nlm.nih.gov | |
Description | Data deposited in or computed by PubChem | |
CAS No. |
354-34-7 | |
Record name | Trifluoroacetyl fluoride | |
Source | CAS Common Chemistry | |
URL | https://commonchemistry.cas.org/detail?cas_rn=354-34-7 | |
Description | CAS Common Chemistry is an open community resource for accessing chemical information. Nearly 500,000 chemical substances from CAS REGISTRY cover areas of community interest, including common and frequently regulated chemicals, and those relevant to high school and undergraduate chemistry classes. This chemical information, curated by our expert scientists, is provided in alignment with our mission as a division of the American Chemical Society. | |
Explanation | The data from CAS Common Chemistry is provided under a CC-BY-NC 4.0 license, unless otherwise stated. | |
Record name | Trifluoroacetyl fluoride | |
Source | ChemIDplus | |
URL | https://pubchem.ncbi.nlm.nih.gov/substance/?source=chemidplus&sourceid=0000354347 | |
Description | ChemIDplus is a free, web search system that provides access to the structure and nomenclature authority files used for the identification of chemical substances cited in National Library of Medicine (NLM) databases, including the TOXNET system. | |
Record name | Acetyl fluoride, 2,2,2-trifluoro- | |
Source | EPA Chemicals under the TSCA | |
URL | https://www.epa.gov/chemicals-under-tsca | |
Description | EPA Chemicals under the Toxic Substances Control Act (TSCA) collection contains information on chemicals and their regulations under TSCA, including non-confidential content from the TSCA Chemical Substance Inventory and Chemical Data Reporting. | |
Record name | Perfluoroacetyl fluoride | |
Source | EPA DSSTox | |
URL | https://comptox.epa.gov/dashboard/DTXSID6059867 | |
Description | DSSTox provides a high quality public chemistry resource for supporting improved predictive toxicology. | |
Record name | Perfluoroacetyl fluoride | |
Source | European Chemicals Agency (ECHA) | |
URL | https://echa.europa.eu/substance-information/-/substanceinfo/100.005.963 | |
Description | The European Chemicals Agency (ECHA) is an agency of the European Union which is the driving force among regulatory authorities in implementing the EU's groundbreaking chemicals legislation for the benefit of human health and the environment as well as for innovation and competitiveness. | |
Explanation | Use of the information, documents and data from the ECHA website is subject to the terms and conditions of this Legal Notice, and subject to other binding limitations provided for under applicable law, the information, documents and data made available on the ECHA website may be reproduced, distributed and/or used, totally or in part, for non-commercial purposes provided that ECHA is acknowledged as the source: "Source: European Chemicals Agency, http://echa.europa.eu/". Such acknowledgement must be included in each copy of the material. ECHA permits and encourages organisations and individuals to create links to the ECHA website under the following cumulative conditions: Links can only be made to webpages that provide a link to the Legal Notice page. | |
Retrosynthesis Analysis
AI-Powered Synthesis Planning: Our tool employs the Template_relevance Pistachio, Template_relevance Bkms_metabolic, Template_relevance Pistachio_ringbreaker, Template_relevance Reaxys, Template_relevance Reaxys_biocatalysis model, leveraging a vast database of chemical reactions to predict feasible synthetic routes.
One-Step Synthesis Focus: Specifically designed for one-step synthesis, it provides concise and direct routes for your target compounds, streamlining the synthesis process.
Accurate Predictions: Utilizing the extensive PISTACHIO, BKMS_METABOLIC, PISTACHIO_RINGBREAKER, REAXYS, REAXYS_BIOCATALYSIS database, our tool offers high-accuracy predictions, reflecting the latest in chemical research and data.
Strategy Settings
Precursor scoring | Relevance Heuristic |
---|---|
Min. plausibility | 0.01 |
Model | Template_relevance |
Template Set | Pistachio/Bkms_metabolic/Pistachio_ringbreaker/Reaxys/Reaxys_biocatalysis |
Top-N result to add to graph | 6 |
Feasible Synthetic Routes
Disclaimer and Information on In-Vitro Research Products
Please be aware that all articles and product information presented on BenchChem are intended solely for informational purposes. The products available for purchase on BenchChem are specifically designed for in-vitro studies, which are conducted outside of living organisms. In-vitro studies, derived from the Latin term "in glass," involve experiments performed in controlled laboratory settings using cells or tissues. It is important to note that these products are not categorized as medicines or drugs, and they have not received approval from the FDA for the prevention, treatment, or cure of any medical condition, ailment, or disease. We must emphasize that any form of bodily introduction of these products into humans or animals is strictly prohibited by law. It is essential to adhere to these guidelines to ensure compliance with legal and ethical standards in research and experimentation.