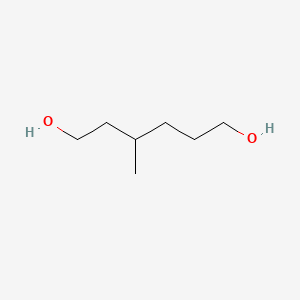
1,6-Hexanediol, 3-methyl-
- Click on QUICK INQUIRY to receive a quote from our team of experts.
- With the quality product at a COMPETITIVE price, you can focus more on your research.
Overview
Description
1,6-Hexanediol, 3-methyl- is an organic compound with the molecular formula C₇H₁₆O₂. It is a diol, meaning it contains two hydroxyl groups (-OH) attached to a hexane backbone. This compound is used in various industrial applications due to its unique chemical properties.
Preparation Methods
Synthetic Routes and Reaction Conditions
1,6-Hexanediol, 3-methyl- can be synthesized through several methods. One common method involves the hydrogenation of dimethyl adipate using supported rhodium-tin catalysts . The reaction typically occurs under high pressure and temperature conditions to ensure complete hydrogenation.
Industrial Production Methods
In industrial settings, 1,6-Hexanediol, 3-methyl- is produced through the catalytic hydrogenation of adipic acid esters. This process involves the use of metal catalysts such as palladium or platinum to facilitate the hydrogenation reaction. The reaction is carried out in a high-pressure reactor to achieve high yields.
Chemical Reactions Analysis
Types of Reactions
1,6-Hexanediol, 3-methyl- undergoes various chemical reactions, including:
Oxidation: The hydroxyl groups can be oxidized to form corresponding aldehydes or carboxylic acids.
Reduction: The compound can be reduced to form alkanes.
Substitution: The hydroxyl groups can be substituted with other functional groups such as halides or esters.
Common Reagents and Conditions
Oxidation: Pyridinium chlorochromate (PCC) is commonly used as an oxidizing agent.
Reduction: Lithium aluminum hydride (LiAlH₄) is often used for reduction reactions.
Substitution: Thionyl chloride (SOCl₂) can be used to substitute hydroxyl groups with chlorine atoms.
Major Products Formed
Oxidation: Adipaldehyde or adipic acid.
Reduction: Hexane.
Substitution: 1,6-Dichloro-3-methylhexane.
Scientific Research Applications
1,6-Hexanediol, 3-methyl- has several applications in scientific research:
Mechanism of Action
The mechanism of action of 1,6-Hexanediol, 3-methyl- involves its ability to act as a crosslinking agent. The hydroxyl groups can form hydrogen bonds with other molecules, facilitating the formation of stable polymer networks. This property is particularly useful in the production of gel polymer electrolytes for batteries .
Comparison with Similar Compounds
Similar Compounds
1,6-Hexanediol: Similar to 1,6-Hexanediol, 3-methyl-, but without the methyl group.
Uniqueness
1,6-Hexanediol, 3-methyl- is unique due to the presence of the methyl group, which can influence its reactivity and physical properties. This makes it particularly useful in applications where specific chemical properties are required.
Properties
IUPAC Name |
3-methylhexane-1,6-diol |
Source
|
---|---|---|
Details | Computed by Lexichem TK 2.7.0 (PubChem release 2021.10.14) | |
Source | PubChem | |
URL | https://pubchem.ncbi.nlm.nih.gov | |
Description | Data deposited in or computed by PubChem | |
InChI |
InChI=1S/C7H16O2/c1-7(4-6-9)3-2-5-8/h7-9H,2-6H2,1H3 |
Source
|
Details | Computed by InChI 1.0.6 (PubChem release 2021.10.14) | |
Source | PubChem | |
URL | https://pubchem.ncbi.nlm.nih.gov | |
Description | Data deposited in or computed by PubChem | |
InChI Key |
SQAJRDHPLTWZQT-UHFFFAOYSA-N |
Source
|
Details | Computed by InChI 1.0.6 (PubChem release 2021.10.14) | |
Source | PubChem | |
URL | https://pubchem.ncbi.nlm.nih.gov | |
Description | Data deposited in or computed by PubChem | |
Canonical SMILES |
CC(CCCO)CCO |
Source
|
Details | Computed by OEChem 2.3.0 (PubChem release 2021.10.14) | |
Source | PubChem | |
URL | https://pubchem.ncbi.nlm.nih.gov | |
Description | Data deposited in or computed by PubChem | |
Molecular Formula |
C7H16O2 |
Source
|
Details | Computed by PubChem 2.2 (PubChem release 2021.10.14) | |
Source | PubChem | |
URL | https://pubchem.ncbi.nlm.nih.gov | |
Description | Data deposited in or computed by PubChem | |
Molecular Weight |
132.20 g/mol |
Source
|
Details | Computed by PubChem 2.2 (PubChem release 2021.10.14) | |
Source | PubChem | |
URL | https://pubchem.ncbi.nlm.nih.gov | |
Description | Data deposited in or computed by PubChem | |
Disclaimer and Information on In-Vitro Research Products
Please be aware that all articles and product information presented on BenchChem are intended solely for informational purposes. The products available for purchase on BenchChem are specifically designed for in-vitro studies, which are conducted outside of living organisms. In-vitro studies, derived from the Latin term "in glass," involve experiments performed in controlled laboratory settings using cells or tissues. It is important to note that these products are not categorized as medicines or drugs, and they have not received approval from the FDA for the prevention, treatment, or cure of any medical condition, ailment, or disease. We must emphasize that any form of bodily introduction of these products into humans or animals is strictly prohibited by law. It is essential to adhere to these guidelines to ensure compliance with legal and ethical standards in research and experimentation.