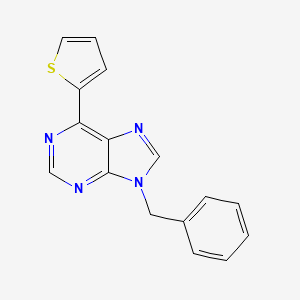
9H-Purine, 9-(phenylmethyl)-6-(2-thienyl)-
- Click on QUICK INQUIRY to receive a quote from our team of experts.
- With the quality product at a COMPETITIVE price, you can focus more on your research.
Overview
Description
9-Benzyl-6-(thiophen-2-yl)-9H-purine is a heterocyclic compound that features a purine core substituted with a benzyl group at the 9-position and a thiophen-2-yl group at the 6-position
Preparation Methods
Synthetic Routes and Reaction Conditions: The synthesis of 9-Benzyl-6-(thiophen-2-yl)-9H-purine typically involves multi-step organic reactions. One common method includes the following steps:
Formation of the Purine Core: The purine core can be synthesized through a condensation reaction between formamide and a suitable aldehyde.
Substitution at the 6-Position: The thiophen-2-yl group can be introduced via a nucleophilic substitution reaction using thiophene-2-boronic acid and a suitable catalyst.
Benzylation at the 9-Position: The benzyl group can be added through a benzylation reaction using benzyl bromide and a base such as potassium carbonate.
Industrial Production Methods: Industrial production of 9-Benzyl-6-(thiophen-2-yl)-9H-purine may involve similar synthetic routes but optimized for large-scale production. This includes the use of continuous flow reactors and automated synthesis equipment to ensure high yield and purity.
Types of Reactions:
Oxidation: The compound can undergo oxidation reactions, particularly at the thiophen-2-yl group, leading to the formation of sulfoxides or sulfones.
Reduction: Reduction reactions can target the purine core or the thiophen-2-yl group, potentially leading to the formation of dihydropurine derivatives.
Substitution: The benzyl and thiophen-2-yl groups can be substituted with other functional groups through nucleophilic or electrophilic substitution reactions.
Common Reagents and Conditions:
Oxidation: Reagents such as hydrogen peroxide or m-chloroperbenzoic acid (m-CPBA) under mild conditions.
Reduction: Reagents like sodium borohydride or lithium aluminum hydride in anhydrous solvents.
Substitution: Halogenated reagents (e.g., benzyl bromide) and bases (e.g., potassium carbonate) in polar aprotic solvents.
Major Products:
Oxidation Products: Sulfoxides and sulfones.
Reduction Products: Dihydropurine derivatives.
Substitution Products: Various substituted purine derivatives depending on the reagents used.
Scientific Research Applications
9-Benzyl-6-(thiophen-2-yl)-9H-purine has several applications in scientific research:
Chemistry: Used as a building block for the synthesis of more complex heterocyclic compounds.
Biology: Investigated for its potential as a kinase inhibitor, which could be useful in studying cell signaling pathways.
Medicine: Explored for its potential therapeutic properties, including anti-cancer and anti-inflammatory activities.
Industry: Utilized in the development of novel materials with specific electronic or photonic properties.
Mechanism of Action
The mechanism of action of 9-Benzyl-6-(thiophen-2-yl)-9H-purine involves its interaction with specific molecular targets, such as kinases. The compound can bind to the active site of the kinase, inhibiting its activity and thereby affecting downstream signaling pathways. This inhibition can lead to various biological effects, including the suppression of cell proliferation in cancer cells.
Comparison with Similar Compounds
6-Benzyl-9H-purine: Lacks the thiophen-2-yl group, making it less versatile in terms of chemical reactivity.
9-Benzyl-6-(furan-2-yl)-9H-purine: Contains a furan ring instead of a thiophene ring, which can affect its electronic properties and reactivity.
9-Benzyl-6-(pyridin-2-yl)-9H-purine: Contains a pyridine ring, which can influence its binding affinity to biological targets.
Uniqueness: 9-Benzyl-6-(thiophen-2-yl)-9H-purine is unique due to the presence of both benzyl and thiophen-2-yl groups, which confer distinct electronic and steric properties. These features make it a valuable compound for various applications in chemistry, biology, and material science.
Biological Activity
9H-Purine, 9-(phenylmethyl)-6-(2-thienyl)- is a synthetic compound belonging to the purine family. Its structure features a purine base with specific substituents at the 9 and 6 positions, namely a phenylmethyl group and a thienyl group. This unique configuration contributes to its potential biological activities, particularly in the fields of antimicrobial and anticancer research.
The compound's reactivity is influenced by the functional groups present on the purine ring. The presence of the phenylmethyl and thienyl groups enhances its chemical properties, allowing it to undergo various transformations and interactions that are critical for its biological activity.
Antimycobacterial Activity
Research indicates that derivatives of purines, including this compound, exhibit significant antimycobacterial activity against Mycobacterium tuberculosis. Structural features such as the thienyl group have been shown to improve efficacy compared to other substituents, making these compounds promising candidates for developing new antimycobacterial agents.
Anticancer Potential
The compound has also been explored for its potential as an inhibitor of topoisomerase II , an enzyme crucial for DNA replication and repair. Inhibition of this enzyme is a well-established strategy in cancer therapy. Studies have demonstrated that purine derivatives can effectively suppress cancer cell growth, with certain analogs showing higher cytotoxicity than established treatments like 5-Fluorouracil and Fludarabine .
Comparative Analysis of Related Compounds
The biological activity of 9H-Purine, 9-(phenylmethyl)-6-(2-thienyl)- can be contextualized by comparing it with other related purine derivatives:
Compound Name | Structure Features | Biological Activity |
---|---|---|
9H-Purine-2,6-diamine | Two amino groups at positions 2 and 6 | Anticancer properties; topoisomerase inhibitor |
N-(4-(7-methyl-7H-purin-6-yl)benzyl) sulfamide | Aryl substituent at position 7 | ENPP1 inhibitor; involved in metabolic regulation |
6-Arylpurines | Various aryl groups at position 6 | Antimycobacterial activity; structure-dependent efficacy |
The table illustrates how the specific combination of substituents in 9H-Purine, 9-(phenylmethyl)-6-(2-thienyl)- enhances its biological activities compared to other derivatives.
Study on Cytotoxicity
In a recent study evaluating various purine derivatives against human cancer cell lines (Huh7, HCT116, MCF7), compounds similar to 9H-Purine were tested using the SRB assay. Notably, certain derivatives exhibited lower IC50 values than standard chemotherapy agents. For example:
Compound | IC50 (μM) against Huh7 Cells | IC50 (μM) against MCF7 Cells |
---|---|---|
Compound A | 14.2 | 30.6 |
Fludarabine | 28.4 | 28.4 |
Cladribine | <0.1 | 2.4 |
This data highlights the potential of specific purine analogs in providing effective alternatives to conventional cancer treatments .
The mechanism by which 9H-Purine, 9-(phenylmethyl)-6-(2-thienyl)- exerts its biological effects involves binding affinities to target enzymes like topoisomerase II. The structural modifications imparted by the phenylmethyl and thienyl groups facilitate enhanced interaction with these targets, leading to improved therapeutic outcomes.
Properties
CAS No. |
160516-05-2 |
---|---|
Molecular Formula |
C16H12N4S |
Molecular Weight |
292.4 g/mol |
IUPAC Name |
9-benzyl-6-thiophen-2-ylpurine |
InChI |
InChI=1S/C16H12N4S/c1-2-5-12(6-3-1)9-20-11-19-15-14(13-7-4-8-21-13)17-10-18-16(15)20/h1-8,10-11H,9H2 |
InChI Key |
PAAXDEJAQCUKRO-UHFFFAOYSA-N |
Canonical SMILES |
C1=CC=C(C=C1)CN2C=NC3=C(N=CN=C32)C4=CC=CS4 |
Origin of Product |
United States |
Disclaimer and Information on In-Vitro Research Products
Please be aware that all articles and product information presented on BenchChem are intended solely for informational purposes. The products available for purchase on BenchChem are specifically designed for in-vitro studies, which are conducted outside of living organisms. In-vitro studies, derived from the Latin term "in glass," involve experiments performed in controlled laboratory settings using cells or tissues. It is important to note that these products are not categorized as medicines or drugs, and they have not received approval from the FDA for the prevention, treatment, or cure of any medical condition, ailment, or disease. We must emphasize that any form of bodily introduction of these products into humans or animals is strictly prohibited by law. It is essential to adhere to these guidelines to ensure compliance with legal and ethical standards in research and experimentation.