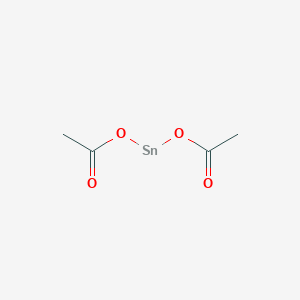
Tin(II) acetate
Overview
Description
It has the chemical formula Sn(CH₃COO)₂ and was first discovered in 1822 . This compound appears as white crystals and is known for its various applications in scientific research and industry.
Mechanism of Action
Target of Action
Tin(II) acetate, with the chemical formula of Sn(CH3COO)2, is the acetate salt of tin(II) It has been used as a catalyst in various chemical reactions .
Mode of Action
this compound can act as a catalyst to enhance the rate of thermal depolymerization of poly(lactic acid) fibers . It can also be a reactant for the synthesis of Sn-Cu bimetallic nanoparticles used in the preparation of tin anode by organic electroplating for rechargeable thin-film batteries .
Biochemical Pathways
It’s known that this compound can be used as a tin source for the preparation of high surface area tin oxide catalysts .
Pharmacokinetics
It’s known that this compound decomposes in water , which could potentially affect its bioavailability.
Result of Action
It’s known that this compound can be used to prepare tin(iv) oxide thin films by photochemical vapor deposition .
Biochemical Analysis
Cellular Effects
It is known that Tin(II) acetate can be used as a catalyst in the synthesis of Sn-Cu bimetallic nanoparticles , which may have implications for cellular function
Molecular Mechanism
It is known that this compound can improve the crystallinity and stability of tin perovskite with fewer defects , which may have implications for its interactions with biomolecules
Temporal Effects in Laboratory Settings
It is known that this compound undergoes disproportionation and decomposition when heated under normal pressure
Dosage Effects in Animal Models
It is known that high dose exposure to tin or tin compounds is harmful to animals
Metabolic Pathways
It is known that acetate and the related metabolism of acetyl-coenzyme A (acetyl-CoA) confer numerous metabolic functions, including energy production, lipid synthesis, and protein acetylation
Preparation Methods
Synthetic Routes and Reaction Conditions: To prepare tin(II) acetate, tin(II) oxide is dissolved in glacial acetic acid and refluxed. This process yields yellow Sn(CH₃COO)₂·2CH₃COOH when cooled. The acetic acid can be removed by heating under reduced pressure, resulting in white Sn(CH₃COO)₂ crystals obtained by sublimation .
Industrial Production Methods: In industrial settings, the preparation of this compound follows similar methods but on a larger scale. The use of high-purity tin(II) oxide and controlled reaction conditions ensures the consistent quality of the final product.
Types of Reactions:
Oxidation: this compound can undergo oxidation to form tin(IV) compounds.
Reduction: It can be reduced to elemental tin under specific conditions.
Substitution: this compound can participate in substitution reactions, where the acetate groups are replaced by other ligands.
Common Reagents and Conditions:
Oxidizing Agents: Hydrogen peroxide or nitric acid can oxidize this compound.
Reducing Agents: Hydrogen gas or sodium borohydride can reduce this compound.
Substitution Reagents: Various organic ligands can replace the acetate groups under appropriate conditions.
Major Products:
Oxidation: Tin(IV) oxide and other tin(IV) compounds.
Reduction: Elemental tin.
Substitution: Complexes with different organic ligands.
Scientific Research Applications
Tin(II) acetate has a wide range of applications in scientific research:
Comparison with Similar Compounds
- Tin(II) chloride (SnCl₂)
- Tin(II) sulfate (SnSO₄)
- Tin(II) oxalate (SnC₂O₄)
- Tin(II) 2-ethylhexanoate (Sn(C₈H₁₅O₂)₂)
Comparison:
- Tin(II) chloride: More soluble in water and commonly used in electroplating.
- Tin(II) sulfate: Used in the preparation of tin-based catalysts and as a reducing agent.
- Tin(II) oxalate: Utilized in the synthesis of tin nanoparticles with specific properties.
- Tin(II) 2-ethylhexanoate: Preferred in organic synthesis due to its solubility in organic solvents.
Tin(II) acetate is unique due to its versatility as a catalyst and its ability to form complexes with various ligands, making it valuable in both research and industrial applications.
Biological Activity
Tin(II) acetate, also known as stannous acetate, is an organotin compound with significant biological activity and diverse applications in various fields, including catalysis, material science, and biological research. Its chemical formula is Sn(CH₃COO)₂, and it appears as a white to off-white crystalline powder. This article explores the biological activity of this compound, its mechanisms of action, and relevant case studies.
This compound has a molecular weight of approximately 236.8 g/mol and a melting point of 182.5-183°C. It is hygroscopic, decomposing in water but soluble in dilute hydrochloric acid, dimethyl sulfoxide, and methanol . The structure consists of tin ions coordinated with acetate ligands, which influence its reactivity and biological interactions .
Catalytic Properties
This compound exhibits Lewis acid properties, making it a valuable catalyst in various organic reactions such as esterification and transesterification. Its catalytic role extends to enhancing the thermal depolymerization of poly(lactic acid) fibers, which has implications in polymer chemistry and material science .
Biological Interactions
Research indicates that this compound can interact with biological systems in several ways:
- Antimicrobial Activity : Organotin compounds, including this compound, have shown antimicrobial properties against various pathogens. Studies have demonstrated the potential of tin complexes to inhibit bacterial growth and exhibit antifungal activity .
- Cellular Effects : this compound has been implicated in cellular processes such as apoptosis induction in cancer cells. Certain studies suggest that organotin compounds can influence cell cycle progression and induce apoptosis through mechanisms involving oxidative stress and mitochondrial dysfunction .
- Microbial Electrosynthesis : In microbial electrosynthesis systems, tin ions from this compound have been shown to enhance methane production while affecting the accumulation of acetate. This suggests a role in modifying microbial metabolic pathways .
1. Antiproliferative Effects
A study investigated the antiproliferative activity of novel organotin compounds, including those derived from this compound. The results indicated that these compounds could block cell proliferation at specific phases of the cell cycle (G2/M phase), highlighting their potential as therapeutic agents against cancer .
2. Electrochemical Applications
In a recent experiment involving microbial electrosynthesis cells (MESCs), the addition of tin ions significantly enhanced methane production while reducing acetate levels. This demonstrates the utility of this compound in biotechnological applications aimed at renewable energy production through microbial processes .
Comparative Analysis
The following table summarizes key features of this compound compared to other organotin compounds:
Compound | Molecular Formula | Unique Features |
---|---|---|
This compound | Sn(CH₃COO)₂ | Lewis acid catalyst; antimicrobial properties |
Tin(IV) Oxide | SnO₂ | Stable oxide form used in electronics |
Trimethylstannane | C₃H₉Sn | Volatile organotin compound used in organic synthesis |
Dibutyltin Dichloride | C₈H₁₈Cl₂Sn | Used as a biocide and stabilizer in plastics |
Safety Considerations
While this compound has beneficial applications, it is essential to note its potential toxicity at high doses. Exposure to tin compounds can be harmful to animals, necessitating careful handling and safety measures during use .
Properties
IUPAC Name |
tin(2+);diacetate | |
---|---|---|
Source | PubChem | |
URL | https://pubchem.ncbi.nlm.nih.gov | |
Description | Data deposited in or computed by PubChem | |
InChI |
InChI=1S/2C2H4O2.Sn/c2*1-2(3)4;/h2*1H3,(H,3,4);/q;;+2/p-2 | |
Source | PubChem | |
URL | https://pubchem.ncbi.nlm.nih.gov | |
Description | Data deposited in or computed by PubChem | |
InChI Key |
PNOXNTGLSKTMQO-UHFFFAOYSA-L | |
Source | PubChem | |
URL | https://pubchem.ncbi.nlm.nih.gov | |
Description | Data deposited in or computed by PubChem | |
Canonical SMILES |
CC(=O)[O-].CC(=O)[O-].[Sn+2] | |
Source | PubChem | |
URL | https://pubchem.ncbi.nlm.nih.gov | |
Description | Data deposited in or computed by PubChem | |
Molecular Formula |
Sn(CH3COO)2, C4H6O4Sn | |
Record name | tin(II) acetate | |
Source | Wikipedia | |
URL | https://en.wikipedia.org/wiki/Dictionary_of_chemical_formulas | |
Description | Chemical information link to Wikipedia. | |
Source | PubChem | |
URL | https://pubchem.ncbi.nlm.nih.gov | |
Description | Data deposited in or computed by PubChem | |
DSSTOX Substance ID |
DTXSID30213242 | |
Record name | Stannous acetate | |
Source | EPA DSSTox | |
URL | https://comptox.epa.gov/dashboard/DTXSID30213242 | |
Description | DSSTox provides a high quality public chemistry resource for supporting improved predictive toxicology. | |
Molecular Weight |
236.80 g/mol | |
Source | PubChem | |
URL | https://pubchem.ncbi.nlm.nih.gov | |
Description | Data deposited in or computed by PubChem | |
CAS No. |
638-39-1 | |
Record name | Stannous acetate | |
Source | ChemIDplus | |
URL | https://pubchem.ncbi.nlm.nih.gov/substance/?source=chemidplus&sourceid=0000638391 | |
Description | ChemIDplus is a free, web search system that provides access to the structure and nomenclature authority files used for the identification of chemical substances cited in National Library of Medicine (NLM) databases, including the TOXNET system. | |
Record name | Stannous acetate | |
Source | EPA DSSTox | |
URL | https://comptox.epa.gov/dashboard/DTXSID30213242 | |
Description | DSSTox provides a high quality public chemistry resource for supporting improved predictive toxicology. | |
Record name | Tin di(acetate) | |
Source | European Chemicals Agency (ECHA) | |
URL | https://echa.europa.eu/substance-information/-/substanceinfo/100.010.306 | |
Description | The European Chemicals Agency (ECHA) is an agency of the European Union which is the driving force among regulatory authorities in implementing the EU's groundbreaking chemicals legislation for the benefit of human health and the environment as well as for innovation and competitiveness. | |
Explanation | Use of the information, documents and data from the ECHA website is subject to the terms and conditions of this Legal Notice, and subject to other binding limitations provided for under applicable law, the information, documents and data made available on the ECHA website may be reproduced, distributed and/or used, totally or in part, for non-commercial purposes provided that ECHA is acknowledged as the source: "Source: European Chemicals Agency, http://echa.europa.eu/". Such acknowledgement must be included in each copy of the material. ECHA permits and encourages organisations and individuals to create links to the ECHA website under the following cumulative conditions: Links can only be made to webpages that provide a link to the Legal Notice page. | |
Record name | STANNOUS ACETATE | |
Source | FDA Global Substance Registration System (GSRS) | |
URL | https://gsrs.ncats.nih.gov/ginas/app/beta/substances/1SKU167W8P | |
Description | The FDA Global Substance Registration System (GSRS) enables the efficient and accurate exchange of information on what substances are in regulated products. Instead of relying on names, which vary across regulatory domains, countries, and regions, the GSRS knowledge base makes it possible for substances to be defined by standardized, scientific descriptions. | |
Explanation | Unless otherwise noted, the contents of the FDA website (www.fda.gov), both text and graphics, are not copyrighted. They are in the public domain and may be republished, reprinted and otherwise used freely by anyone without the need to obtain permission from FDA. Credit to the U.S. Food and Drug Administration as the source is appreciated but not required. | |
Retrosynthesis Analysis
AI-Powered Synthesis Planning: Our tool employs the Template_relevance Pistachio, Template_relevance Bkms_metabolic, Template_relevance Pistachio_ringbreaker, Template_relevance Reaxys, Template_relevance Reaxys_biocatalysis model, leveraging a vast database of chemical reactions to predict feasible synthetic routes.
One-Step Synthesis Focus: Specifically designed for one-step synthesis, it provides concise and direct routes for your target compounds, streamlining the synthesis process.
Accurate Predictions: Utilizing the extensive PISTACHIO, BKMS_METABOLIC, PISTACHIO_RINGBREAKER, REAXYS, REAXYS_BIOCATALYSIS database, our tool offers high-accuracy predictions, reflecting the latest in chemical research and data.
Strategy Settings
Precursor scoring | Relevance Heuristic |
---|---|
Min. plausibility | 0.01 |
Model | Template_relevance |
Template Set | Pistachio/Bkms_metabolic/Pistachio_ringbreaker/Reaxys/Reaxys_biocatalysis |
Top-N result to add to graph | 6 |
Feasible Synthetic Routes
A: Tin(II) acetate exists as a highly distorted trigonal bipyramid in the gas phase. [] The tin atom is asymmetrically bonded to two acetate groups, with one Sn-O bond significantly longer than the other. [] This asymmetry arises from the lone pair of electrons on the tin atom, which occupies one of the equatorial sites in the trigonal bipyramidal geometry. [] The four-membered rings and the acetate groups themselves adopt a near-planar arrangement. []
A: Yes, this compound is a valuable precursor in material science. For instance, it's used to synthesize tin sulfide nanosheets. [] By controlling the ligands during synthesis, researchers can tune the morphology of the resulting nanosheets, influencing their lateral dimensions and thicknesses. [] This control over morphology is crucial for optimizing the material's properties for applications like photo-detection and photovoltaics. []
A: this compound is a key ingredient in creating fluorine-doped tin dioxide thin films, crucial components in many electronic devices. [] When this compound undergoes chemical vapor deposition, it efficiently incorporates fluorine atoms into the tin dioxide crystal structure. [] This doping increases carrier concentration, leading to films with exceptionally low resistivity. [] Such films are highly desirable for their transparent and conductive properties, finding applications in displays, solar cells, and other optoelectronic devices. []
A: Absolutely, this compound serves as a precursor for synthesizing various nanoparticles, including silver and tin nanoparticles. [, , ] In the case of silver nanoparticles, this compound acts as a reducing agent, facilitating the reduction of silver ions to silver atoms. [] This process, often carried out in the presence of a capping agent like dodecylamine, yields nanoparticles with controlled sizes suitable for applications like conductive ink formulations in printed electronics. [] The size and shape of tin nanoparticles can be influenced by factors like the molecular weight of capping agents such as polyvinyl pyrrolidone (PVP). []
A: this compound demonstrates catalytic activity in various organic reactions. For example, it acts as a Lewis acid catalyst in the reaction between 3-aminopyrrole and trifluoromethyl-β-diketones. [] Its presence influences the regiochemistry of the reaction, favoring the formation of the α-1H-pyrrolo[3,2-b]pyridine isomer. [] This regioselectivity is crucial for synthesizing specific isomers with desired biological or pharmaceutical properties. Furthermore, this compound, along with tin(IV) acetate, dibutyltin-bis-acetate, and dioctyl tin-bis-acetate, can catalyze the ring-opening polymerization of L-lactide and ε-caprolactone. [] The specific tin compound and reaction conditions significantly impact the resulting polymer architecture, enabling the synthesis of linear chains or cyclic structures with varying molecular weights. []
A: this compound acts as a catalyst, significantly accelerating the degradation of PLA. [] Compared to other organometallic catalysts like tin(II) oxalate, this compound demonstrates superior performance, lowering the degradation onset temperature of PLA and drastically reducing the total degradation time. [] This catalytic activity is particularly relevant in developing efficient methods for PLA degradation, addressing its disposal and recycling challenges.
A: this compound plays a crucial role as an additive in the fabrication of tin-lead-based perovskite solar cells. [] Its presence enhances the stability and performance of these devices. While the specific mechanisms are still under investigation, research suggests that this compound influences the crystallization process during perovskite film formation, leading to improved film quality and device stability. []
A: While this compound has shown promising applications, its safety profile requires careful consideration. In vitro studies on human lymphocytes have shown that while this compound does not induce micronuclei formation, indicating a lack of genotoxic effects, it does exhibit cytotoxicity at certain concentrations. [] These findings highlight the importance of handling this compound with caution and using appropriate safety measures during synthesis, handling, and disposal.
A: Researchers utilize a variety of analytical techniques to characterize and study this compound. X-ray diffraction is crucial for determining its crystal structure. [, ] Electron diffraction, coupled with ab initio calculations, provides insights into its gas-phase structure. [] Infrared spectroscopy helps identify functional groups and study chemical bonding. [] Thermal analysis methods like thermogravimetric analysis (TGA) are essential for studying its thermal stability and decomposition behavior. []
Disclaimer and Information on In-Vitro Research Products
Please be aware that all articles and product information presented on BenchChem are intended solely for informational purposes. The products available for purchase on BenchChem are specifically designed for in-vitro studies, which are conducted outside of living organisms. In-vitro studies, derived from the Latin term "in glass," involve experiments performed in controlled laboratory settings using cells or tissues. It is important to note that these products are not categorized as medicines or drugs, and they have not received approval from the FDA for the prevention, treatment, or cure of any medical condition, ailment, or disease. We must emphasize that any form of bodily introduction of these products into humans or animals is strictly prohibited by law. It is essential to adhere to these guidelines to ensure compliance with legal and ethical standards in research and experimentation.