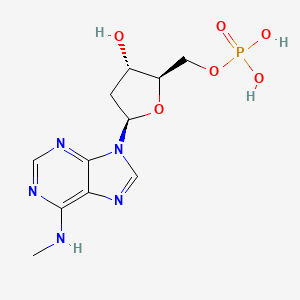
N6-Methyl-deoxy-adenosine-5'-monophosphate
- Click on QUICK INQUIRY to receive a quote from our team of experts.
- With the quality product at a COMPETITIVE price, you can focus more on your research.
Overview
Description
N6-Methyl-deoxy-adenosine-5’-monophosphate is a modified nucleotide that plays a significant role in various biological processes. It is a derivative of adenosine monophosphate where a methyl group is added to the nitrogen at the sixth position of the adenine base. This modification is known to influence gene expression and stability of nucleic acids .
Preparation Methods
Synthetic Routes and Reaction Conditions
The synthesis of N6-Methyl-deoxy-adenosine-5’-monophosphate typically involves the methylation of deoxyadenosine monophosphate. This can be achieved using methylating agents such as methyl iodide or dimethyl sulfate under controlled conditions. The reaction is usually carried out in an aqueous solution with a suitable base to neutralize the acid formed during the reaction .
Industrial Production Methods
Industrial production of N6-Methyl-deoxy-adenosine-5’-monophosphate involves large-scale methylation processes. These processes are optimized for high yield and purity, often using automated systems to control reaction parameters such as temperature, pH, and concentration of reactants. The product is then purified using techniques like high-performance liquid chromatography (HPLC) to ensure it meets the required specifications .
Chemical Reactions Analysis
Types of Reactions
N6-Methyl-deoxy-adenosine-5’-monophosphate can undergo various chemical reactions, including:
Oxidation: This compound can be oxidized to form N6-methyl-deoxy-adenosine-5’-diphosphate and N6-methyl-deoxy-adenosine-5’-triphosphate.
Reduction: Reduction reactions can convert it back to its deoxyadenosine monophosphate form.
Substitution: It can participate in nucleophilic substitution reactions where the methyl group can be replaced by other functional groups.
Common Reagents and Conditions
Common reagents used in these reactions include oxidizing agents like potassium permanganate for oxidation, reducing agents like sodium borohydride for reduction, and nucleophiles like ammonia for substitution reactions. The reactions are typically carried out under mild conditions to prevent degradation of the nucleotide .
Major Products Formed
The major products formed from these reactions include various phosphorylated derivatives of N6-Methyl-deoxy-adenosine, such as N6-methyl-deoxy-adenosine-5’-diphosphate and N6-methyl-deoxy-adenosine-5’-triphosphate .
Scientific Research Applications
N6-Methyl-deoxy-adenosine-5’-monophosphate has a wide range of applications in scientific research:
Chemistry: It is used as a model compound to study methylation effects on nucleotides.
Biology: It plays a role in the regulation of gene expression and is used in studies related to epigenetics.
Medicine: It is investigated for its potential therapeutic applications in treating diseases related to gene expression abnormalities.
Industry: It is used in the production of modified nucleotides for various biotechnological applications
Mechanism of Action
The mechanism of action of N6-Methyl-deoxy-adenosine-5’-monophosphate involves its incorporation into nucleic acids, where it can influence the stability and expression of genes. The methyl group at the sixth position of adenine can affect the binding of transcription factors and other proteins to DNA, thereby regulating gene expression. This modification can also impact the stability of mRNA, influencing its translation efficiency .
Comparison with Similar Compounds
Similar Compounds
N6-Methyladenosine: Similar to N6-Methyl-deoxy-adenosine-5’-monophosphate but lacks the deoxy modification.
5-Methylcytosine: Another methylated nucleotide involved in gene regulation.
7-Methylguanosine: A methylated nucleotide found in the cap structure of eukaryotic mRNA.
Uniqueness
N6-Methyl-deoxy-adenosine-5’-monophosphate is unique due to its specific methylation at the sixth position of adenine and the presence of a deoxy sugar. This combination of modifications makes it a valuable tool for studying the effects of methylation on DNA and RNA stability and function .
Properties
CAS No. |
53696-69-8 |
---|---|
Molecular Formula |
C11H16N5O6P |
Molecular Weight |
345.25 g/mol |
IUPAC Name |
[(2R,3S,5R)-3-hydroxy-5-[6-(methylamino)purin-9-yl]oxolan-2-yl]methyl dihydrogen phosphate |
InChI |
InChI=1S/C11H16N5O6P/c1-12-10-9-11(14-4-13-10)16(5-15-9)8-2-6(17)7(22-8)3-21-23(18,19)20/h4-8,17H,2-3H2,1H3,(H,12,13,14)(H2,18,19,20)/t6-,7+,8+/m0/s1 |
InChI Key |
MGKYNCZAQIZDCV-XLPZGREQSA-N |
Isomeric SMILES |
CNC1=C2C(=NC=N1)N(C=N2)[C@H]3C[C@@H]([C@H](O3)COP(=O)(O)O)O |
Canonical SMILES |
CNC1=C2C(=NC=N1)N(C=N2)C3CC(C(O3)COP(=O)(O)O)O |
Origin of Product |
United States |
Disclaimer and Information on In-Vitro Research Products
Please be aware that all articles and product information presented on BenchChem are intended solely for informational purposes. The products available for purchase on BenchChem are specifically designed for in-vitro studies, which are conducted outside of living organisms. In-vitro studies, derived from the Latin term "in glass," involve experiments performed in controlled laboratory settings using cells or tissues. It is important to note that these products are not categorized as medicines or drugs, and they have not received approval from the FDA for the prevention, treatment, or cure of any medical condition, ailment, or disease. We must emphasize that any form of bodily introduction of these products into humans or animals is strictly prohibited by law. It is essential to adhere to these guidelines to ensure compliance with legal and ethical standards in research and experimentation.