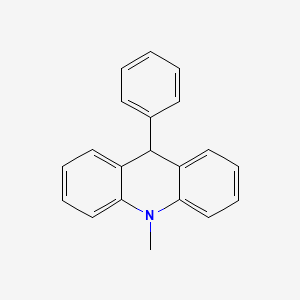
10-Methyl-9-phenyl-9,10-dihydroacridine
- Click on QUICK INQUIRY to receive a quote from our team of experts.
- With the quality product at a COMPETITIVE price, you can focus more on your research.
Overview
Description
10-Methyl-9-phenyl-9,10-dihydroacridine (PhAcrH) is a nitrogen-containing heterocyclic compound characterized by a dihydroacridine core substituted with methyl and phenyl groups at positions 9 and 10, respectively. Its structure enables unique photophysical and redox properties, making it a subject of interest in organic electronics, photochemistry, and materials science. Studies highlight its role in excited-state hydrogen transfer reactions, photo-oxidation processes, and as a donor in thermally activated delayed fluorescence (TADF) systems .
Preparation Methods
Synthetic Routes and Reaction Conditions
The synthesis of 10-Methyl-9-phenyl-9,10-dihydroacridine typically involves the reaction of phenylacetonitrile with N-methylacridone under specific conditions. The reaction is carried out in the presence of a base such as sodium hydride in a solvent like dimethylformamide (DMF). The mixture is then heated to facilitate the formation of the desired product .
Industrial Production Methods
Industrial production methods for this compound are similar to laboratory synthesis but are scaled up to accommodate larger quantities. The reaction conditions are optimized to ensure high yield and purity of the product. The use of continuous flow reactors and automated systems can enhance the efficiency of the production process .
Chemical Reactions Analysis
Types of Reactions
10-Methyl-9-phenyl-9,10-dihydroacridine undergoes various chemical reactions, including:
Oxidation: The compound can be oxidized to form the corresponding iminium ion.
Reduction: It can be reduced to form the corresponding amine.
Substitution: The phenyl and methyl groups can undergo substitution reactions under specific conditions.
Common Reagents and Conditions
Oxidation: Common oxidizing agents include potassium permanganate and hydrogen peroxide.
Reduction: Reducing agents such as lithium aluminum hydride and sodium borohydride are used.
Substitution: Substitution reactions often require catalysts like palladium on carbon and specific solvents like acetonitrile.
Major Products Formed
Oxidation: The major product is the iminium ion.
Reduction: The major product is the corresponding amine.
Substitution: The products depend on the substituents introduced during the reaction.
Scientific Research Applications
Photochemical Applications
1. Chromophores for pOH Jump Experiments
The compound has been studied for its role as a chromophore in photochemical reactions. Specifically, the excited-state behavior of derivatives such as 9-hydroxy-10-methyl-9-phenyl-9,10-dihydroacridine has been investigated using femtosecond and nanosecond UV-vis transient absorption spectroscopy. These studies revealed that the compound exhibits fast heterolytic cleavage in protic solvents, generating long-lived species that can be utilized in pOH jump experiments, making it valuable for understanding reaction dynamics in solution .
2. Organic Photohydrides
Research indicates that 10-methyl-9-phenyl-9,10-dihydroacridine can function as an organic photohydride, where it releases hydride ions upon excitation. This property is particularly significant for applications in dye-sensitized solar cells (DSSCs), where efficient charge transfer and energy conversion are crucial . The excited-state behavior of the compound suggests it could enhance the performance of solar energy devices by improving light absorption and electron transfer processes.
Catalytic Applications
1. Water Oxidation Catalysts
The compound's derivatives have been explored as potential catalysts for water oxidation, a critical process in energy conversion technologies. A study led by Professor Ksenija Glusac demonstrated that iminium ions derived from this compound can act as effective catalysts in the oxidation of water to molecular oxygen. This process involves multiple steps, including the formation of alkoxyl radicals and their subsequent coupling to produce peroxide derivatives . The research highlights the compound's potential in developing sustainable energy solutions.
1. Mechanistic Studies on Photochemical Reactions
A detailed mechanistic study published in The Journal of Physical Chemistry B examined the photochemical behavior of this compound derivatives. The research focused on solvent effects on C-O bond cleavage, revealing insights into how different environments influence reaction kinetics .
2. Development of Organic Catalysts
In another notable study, researchers investigated the use of iminium salts derived from this compound as catalysts for water oxidation. The work demonstrated a novel approach to electrocatalytic processes essential for renewable energy applications . The findings contributed significantly to the understanding of structure-property relationships in organic catalysts.
Mechanism of Action
The mechanism of action of 10-Methyl-9-phenyl-9,10-dihydroacridine involves the release of a hydride ion upon excitation. This process occurs through a stepwise electron/hydrogen-atom transfer mechanism. Upon excitation, the compound is oxidized to the corresponding iminium ion, while the solvent is reduced. This mechanism is crucial for its applications in photochemical reactions and hydride transfer processes .
Comparison with Similar Compounds
Structural Analogues and Substituent Effects
PhAcrH is part of a broader class of 9,10-dihydroacridine derivatives. Key structural analogues include:
- 9,9-Dimethyl-9,10-dihydroacridine (DMAC) : Lacks the phenyl group but has two methyl substituents at position 7.
- 9,9-Diphenyl-9,10-dihydroacridine (DPAC) : Features phenyl groups at both positions 9 and 10.
Substituent Impact on Properties :
- Photo-Oxidation Efficiency : PhAcrH exhibits a 93% yield in photo-oxidation to acridone derivatives under neutral conditions, outperforming DMAC (91%) and DPAC (82% with benzoyl substituents) .
- Synthetic Reactivity : Bulky substituents (e.g., tert-butoxycarbonyl) enhance reactivity in cross-coupling reactions, as seen in the synthesis of ester-substituted dihydroacridines .
Photophysical and TADF Properties
Table 1 summarizes key photophysical parameters:
- TADF Performance: DMAC derivatives exhibit lower ΔEST (0.06 eV) due to stronger donor-acceptor separation, enabling efficient reverse intersystem crossing (RISC). PhAcrH’s higher ΔEST (0.18–0.43 eV) limits TADF efficiency but enhances fluorescence in rigid matrices .
- Solvent Polarity Effects : PhAcrH shows red-shifted emission in polar solvents (e.g., DCM), with PLQY increasing from 15% (solution) to 37% (solid state) due to suppressed vibrational quenching .
Electrochemical and Redox Behavior
- Ionization Potentials (IP): PhAcrH and its dimethyl analogue (DMAC) share similar IP values (~5.3 eV), indicating comparable electron-donating strengths .
- Electron Affinity (EA) : Substituents significantly alter EA. For example, DMAC-based DAD compounds show |EA| = 2.59–2.84 eV, whereas phenyl-substituted derivatives (e.g., DPAC) exhibit higher |EA| (~3.0 eV) due to extended conjugation .
Reactivity in Oxidation and Functionalization
- Oxidation to Acridones : PhAcrH undergoes efficient photo-oxidation to acridone derivatives (93% yield), comparable to DMAC (91%) but superior to tert-butyl-protected analogues (89%) .
- Borylation Selectivity : Substituents dictate regioselectivity in electrophilic C–H borylation. For example, 9,9-diphenyl derivatives favor para-borylation, while dimethyl analogues show ortho-selectivity .
Biological Activity
10-Methyl-9-phenyl-9,10-dihydroacridine is a compound belonging to the dihydroacridine class, which is characterized by a fused ring structure that includes an acridine core. This compound has garnered interest due to its unique photophysical properties and potential biological activities. This article explores the biological activity of this compound, including its mechanisms of action, pharmacological effects, and relevant case studies.
The molecular formula of this compound is C20H17N, indicating that it comprises 20 carbon atoms, 17 hydrogen atoms, and one nitrogen atom. The presence of the methyl group at the 10-position and the phenyl group at the 9-position contributes to its distinct chemical reactivity and excited-state dynamics.
Mechanisms of Biological Activity
Research into the biological activity of this compound has revealed several mechanisms through which this compound may exert its effects:
- Photochemical Behavior : Upon excitation, this compound can undergo oxidation to form an iminium ion. This transformation is significant in photochemical studies and may lead to various biological interactions .
- Hydroxide Ion Release : Studies have shown that photochemical reactions involving this compound can generate hydroxide ions. The release of these ions can influence cellular processes and contribute to its biological activity .
- Electron Transfer Mechanisms : The excited state of this compound facilitates electron transfer processes that are essential for its reactivity in biological systems. This property is particularly relevant in the context of oxidative stress and cellular signaling pathways .
Biological Applications
The potential applications of this compound span various fields:
- Pharmaceutical Development : Compounds in the dihydroacridine class are often investigated for their potential as therapeutic agents. Although specific pharmacological effects of this compound are still being explored, related compounds have shown promise in treating neurodegenerative diseases and cancer.
- Organic Photohydrides : The compound's ability to participate in photochemical reactions positions it as a candidate for use in organic photohydride applications, which may have implications for energy storage and conversion technologies .
Case Studies
Several studies have been conducted to elucidate the biological activity of this compound:
- Photochemical Hydroxide Ion Release : A mechanistic study demonstrated that upon UV excitation, this compound releases hydroxide ions efficiently in protic solvents. The reaction kinetics were characterized using transient absorption spectroscopy, revealing fast heterolytic cleavage processes .
- Comparative Studies with Derivatives : Research comparing this compound with its derivatives highlighted differences in reactivity and biological behavior. For instance, derivatives like 9-hydroxy and N,N-dimethylamino-substituted compounds exhibited varying degrees of solubility and biological activity, suggesting that structural modifications can significantly affect their pharmacological profiles .
Data Table: Comparison of Related Compounds
Compound Name | Structural Features | Unique Characteristics |
---|---|---|
This compound | Methyl group at position 10 | Exhibits distinct photochemical behavior and reactivity |
9-Hydroxy-10-methyl-9,10-dihydroacridine | Hydroxy group at position 9 | Demonstrates different solubility and biological activity |
N,N-Dimethylamino-substituted derivatives | Dimethylamino group at various positions | Enhanced solubility and potential biological activity |
Q & A
Basic Research Questions
Q. What are the key synthetic routes for 10-Methyl-9-phenyl-9,10-dihydroacridine derivatives?
The synthesis of derivatives often involves nucleophilic addition or photo-induced coupling. For example, 4,9-dimethyl-9,10-dihydroacridine is synthesized via methyllithium addition to 4-methylacridine in THF, followed by recrystallization in chloroform/ether (yield: 55%) . Photo-induced coupling reactions under λ > 320 nm irradiation in deaerated acetonitrile can generate products like 9-dicyanomethyl-9-(10'-methyl-9'-acridinyl)fluorene, confirmed via X-ray crystallography and NMR .
Q. How are the photophysical properties of this compound derivatives characterized?
Key techniques include:
- Femtosecond/nanosecond transient absorption spectroscopy : To study excited-state dynamics (e.g., heterolytic C–O bond cleavage in protic solvents, τ = 108 ps) .
- UV-vis and fluorescence spectroscopy : To analyze solvent-dependent emission (e.g., orange-red fluorescence in polar solvents) and quantum yields (PLQY up to 37% in toluene) .
- Electrochemical methods : To determine redox potentials (e.g., ionization potentials: 5.28–5.33 eV) and electron affinities .
Advanced Research Questions
Q. How do solvent effects influence the excited-state behavior of this compound derivatives?
Solvent polarity dictates reaction pathways:
- Protic solvents (e.g., water) : Promote fast heterolytic cleavage (108 ps), generating 10-methyl-9-phenylacridinium (Acr⁺) and hydroxide ions (⁻OH) with long recombination lifetimes (>100 μs) .
- Aprotic solvents (e.g., acetonitrile) : Favor intersystem crossing over bond cleavage, altering photochemical outcomes . Methodological Insight: Use time-resolved spectroscopy to monitor solvent-dependent kinetics and transient species.
Q. What computational protocols predict redox properties and hydride transfer energetics in these compounds?
Protocols developed by the Glusac group combine density functional theory (DFT) with experimental validation:
- Calculate Gibbs free energies of hydride transfer with <2 kcal/mol deviation from experimental data .
- Screen redox-active compounds using HOMO/LUMO energies and charge-transfer parameters . Application: These methods enable rapid computational screening of photo-catalysts for solar fuel applications .
Q. How does molecular engineering of donor-acceptor systems impact thermally activated delayed fluorescence (TADF) efficiency?
Structural modifications are critical:
- Rigid donors (e.g., 9,9-dimethyl-9,10-dihydroacridine) : Reduce non-radiative decay via steric hindrance, enhancing PLQY (e.g., 37% in toluene) .
- ΔE(S₁–T₁) tuning : DFT calculations show ΔE(S₁–T₁) = 0.06–0.43 eV, enabling reverse intersystem crossing (RISC) for TADF . Design Strategy: Attach electron-withdrawing groups (e.g., benzothiadiazole) to optimize charge transfer and triplet harvesting .
Q. How can contradictions in solvent-dependent reaction mechanisms be resolved?
Conflicting observations (e.g., bond cleavage vs. intersystem crossing) arise from solvent polarity and proton availability. To resolve:
- Compare transient absorption spectra across solvents .
- Validate computational models (e.g., solvation free energy calculations) against experimental kinetics .
Q. Methodological Recommendations
- For synthesis : Prioritize photo-induced coupling for complex derivatives or nucleophilic addition for methyl-substituted analogs .
- For TADF optimization : Use DFT to predict ΔE(S₁–T₁) and steric effects, then validate with PLQY measurements .
- For mechanistic studies : Combine transient spectroscopy with solvent polarity scales (e.g., ET(30)) to rationalize reaction pathways .
Properties
CAS No. |
56875-26-4 |
---|---|
Molecular Formula |
C20H17N |
Molecular Weight |
271.4 g/mol |
IUPAC Name |
10-methyl-9-phenyl-9H-acridine |
InChI |
InChI=1S/C20H17N/c1-21-18-13-7-5-11-16(18)20(15-9-3-2-4-10-15)17-12-6-8-14-19(17)21/h2-14,20H,1H3 |
InChI Key |
IMVRUPICRVHSAZ-UHFFFAOYSA-N |
Canonical SMILES |
CN1C2=CC=CC=C2C(C3=CC=CC=C31)C4=CC=CC=C4 |
Origin of Product |
United States |
Disclaimer and Information on In-Vitro Research Products
Please be aware that all articles and product information presented on BenchChem are intended solely for informational purposes. The products available for purchase on BenchChem are specifically designed for in-vitro studies, which are conducted outside of living organisms. In-vitro studies, derived from the Latin term "in glass," involve experiments performed in controlled laboratory settings using cells or tissues. It is important to note that these products are not categorized as medicines or drugs, and they have not received approval from the FDA for the prevention, treatment, or cure of any medical condition, ailment, or disease. We must emphasize that any form of bodily introduction of these products into humans or animals is strictly prohibited by law. It is essential to adhere to these guidelines to ensure compliance with legal and ethical standards in research and experimentation.