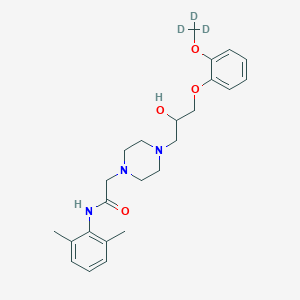
Ranolazine-d3
Overview
Description
Ranolazine is an anti-ischemic agent which modulates myocardial metabolism. Antianginal.
Scientific Research Applications
Electrophysiological Effects
Ranolazine is recognized for its electrophysiological effects and antiarrhythmic actions in both the ventricles and atria. It has shown efficacy in suppressing arrhythmias associated with conditions like acute coronary syndrome, long QT syndrome, heart failure, ischemia, and reperfusion. Notably, it is effective in suppressing atrial tachyarrhythmias and atrial fibrillation (AF). The primary mechanism of its action is believed to be through the inhibition of late I(Na) in the ventricles and use-dependent inhibition of peak I(Na) and I(Kr) in the atria. These findings are supported by a variety of studies and clinical trials (Antzelevitch et al., 2011).
Cardioprotective Properties
Research indicates that ranolazine can protect against ischemia-reperfusion injury in isolated hearts. It achieves this by reducing Ca2+ overload and oxidative stress, and improving mitochondrial integrity. This effect is attributed to its ability to block the late Na+ current during ischemia and modulate mitochondrial metabolism (Aldakkak et al., 2011).
Molecular Basis of Ranolazine Action
Studies have delved into the molecular basis of ranolazine’s action, particularly its effects on sodium channels. Ranolazine has been shown to preferentially block sustained Na+ channel current, especially in long QT syndrome variant 3 (LQT-3) mutant channels. This research highlights the utility of ranolazine as a blocker of sustained Na+ channel activity induced by inherited mutations (Fredj et al., 2006).
Treatment of Diabetic Cardiac Fibrosis
Ranolazine shows potential in treating diabetic cardiac fibrosis, a major cardiovascular complication of diabetes mellitus. It inhibits pyroptosis and collagen deposition, thus improving cardiac function. The mechanism involves upregulation of miR-135b, which plays a role in delaying the progression of numerous cardiovascular diseases (Ren et al., 2022).
Metabolic Effects
Ranolazine stimulates glucose oxidation in both normoxic and ischemic conditions. This property is valuable in the context of cardiac ischemia and reperfusion, as it allows for improved ATP/O2 and reduction in the buildup of harmful byproducts (McCormack et al., 1996).
Additional Therapeutic Applications
Beyond its primary use for chronic angina, ranolazine has been explored for its therapeutic potential in conditions like atrial fibrillation, diabetes, and other cardiovascular diseases. These studies have expanded our understanding of ranolazine’s mechanism of action and its potential applications in different pathological conditions (Banerjee et al., 2017).
Mechanism of Action
Mode of Action
Ranolazine inhibits the late phase of the inward sodium channel during cardiac repolarization . This inhibition reduces intracellular sodium concentrations, thereby reducing calcium influx via the sodium-calcium exchange . Under hypoxic conditions, common to growing tumors, VGSCs develop a persistent current (INaP) which can be blocked selectively by ranolazine .
Biochemical Pathways
Ranolazine affects the biochemical pathways related to energy production in the heart. It prevents fatty acid oxidation and favors glucose utilization, ameliorating the “energy starvation” of the failing heart . This shift in myocardial energy production from fatty acid oxidation to glucose oxidation is a key aspect of ranolazine’s action .
Pharmacokinetics
Ranolazine is extensively metabolized by cytochrome P450 (CYP) 3A enzymes and, to a lesser extent, by CYP2D6 . Approximately 5% is excreted renally unchanged . The elimination half-life of ranolazine is 1.4–1.9 hours . The absolute bioavailability ranges from 35% to 50% . Ranolazine’s pharmacokinetics are unaffected by sex, congestive heart failure, and diabetes mellitus . The area under the curve (auc) increases up to 2-fold with advancing degree of renal impairment .
Result of Action
Ranolazine’s action results in significant molecular and cellular effects. In vitro, ranolazine inhibits the invasiveness of cancer cells, especially under hypoxia . In vivo, it suppresses the metastatic abilities of breast and prostate cancers and melanoma . In the context of heart disease, ranolazine improves myocardial function and perfusion .
Action Environment
The action, efficacy, and stability of ranolazine can be influenced by environmental factors. For instance, under hypoxic conditions common to growing tumors, the drug’s ability to block VGSCs is enhanced . Furthermore, the drug’s effectiveness can be boosted by co-administration with other drugs . .
Safety and Hazards
Biochemical Analysis
Biochemical Properties
Ranolazine-d3, like its parent compound Ranolazine, interacts with various enzymes and proteins. It is known to inhibit late sodium channels, which can lead to beneficial downstream effects . This inhibition reduces intracellular calcium overload, a common occurrence in ischemic cardiomyocytes .
Cellular Effects
This compound has significant effects on various types of cells and cellular processes. It influences cell function by minimizing calcium overload in ischemic cardiomyocytes . This can impact cell signaling pathways, gene expression, and cellular metabolism .
Molecular Mechanism
The molecular mechanism of this compound involves its interaction with biomolecules at the molecular level. It inhibits late sodium current, thereby reducing sodium entry into ischemic myocardial cells . This is proposed to reduce calcium uptake indirectly via the sodium/calcium exchanger, preserving ionic homeostasis and reversing ischemia-induced contractile dysfunction .
Dosage Effects in Animal Models
In animal models, the effects of this compound vary with different dosages. For instance, in a goat model of lone atrial fibrillation, the highest dose of Ranolazine significantly prolonged the atrial effective refractory period and decreased atrial conduction velocity .
Metabolic Pathways
This compound is involved in several metabolic pathways. It is extensively metabolized by cytochrome P450 (CYP) 3A enzymes and, to a lesser extent, by CYP2D6 . It also prevents fatty acid oxidation and favors glucose utilization, ameliorating the “energy starvation” of the failing heart .
Transport and Distribution
It is known that Ranolazine is extensively metabolized by cytochrome P450 (CYP) 3A enzymes and, to a lesser extent, by CYP2D6, with approximately 5% excreted renally unchanged .
Properties
IUPAC Name |
N-(2,6-dimethylphenyl)-2-[4-[2-hydroxy-3-[2-(trideuteriomethoxy)phenoxy]propyl]piperazin-1-yl]acetamide | |
---|---|---|
Source | PubChem | |
URL | https://pubchem.ncbi.nlm.nih.gov | |
Description | Data deposited in or computed by PubChem | |
InChI |
InChI=1S/C24H33N3O4/c1-18-7-6-8-19(2)24(18)25-23(29)16-27-13-11-26(12-14-27)15-20(28)17-31-22-10-5-4-9-21(22)30-3/h4-10,20,28H,11-17H2,1-3H3,(H,25,29)/i3D3 | |
Source | PubChem | |
URL | https://pubchem.ncbi.nlm.nih.gov | |
Description | Data deposited in or computed by PubChem | |
InChI Key |
XKLMZUWKNUAPSZ-HPRDVNIFSA-N | |
Source | PubChem | |
URL | https://pubchem.ncbi.nlm.nih.gov | |
Description | Data deposited in or computed by PubChem | |
Canonical SMILES |
CC1=C(C(=CC=C1)C)NC(=O)CN2CCN(CC2)CC(COC3=CC=CC=C3OC)O | |
Source | PubChem | |
URL | https://pubchem.ncbi.nlm.nih.gov | |
Description | Data deposited in or computed by PubChem | |
Isomeric SMILES |
[2H]C([2H])([2H])OC1=CC=CC=C1OCC(CN2CCN(CC2)CC(=O)NC3=C(C=CC=C3C)C)O | |
Source | PubChem | |
URL | https://pubchem.ncbi.nlm.nih.gov | |
Description | Data deposited in or computed by PubChem | |
Molecular Formula |
C24H33N3O4 | |
Source | PubChem | |
URL | https://pubchem.ncbi.nlm.nih.gov | |
Description | Data deposited in or computed by PubChem | |
DSSTOX Substance ID |
DTXSID60648922 | |
Record name | N-(2,6-Dimethylphenyl)-2-[4-(2-hydroxy-3-{2-[(~2~H_3_)methyloxy]phenoxy}propyl)piperazin-1-yl]acetamide | |
Source | EPA DSSTox | |
URL | https://comptox.epa.gov/dashboard/DTXSID60648922 | |
Description | DSSTox provides a high quality public chemistry resource for supporting improved predictive toxicology. | |
Molecular Weight |
430.6 g/mol | |
Source | PubChem | |
URL | https://pubchem.ncbi.nlm.nih.gov | |
Description | Data deposited in or computed by PubChem | |
CAS No. |
1054624-77-9 | |
Record name | N-(2,6-Dimethylphenyl)-2-[4-(2-hydroxy-3-{2-[(~2~H_3_)methyloxy]phenoxy}propyl)piperazin-1-yl]acetamide | |
Source | EPA DSSTox | |
URL | https://comptox.epa.gov/dashboard/DTXSID60648922 | |
Description | DSSTox provides a high quality public chemistry resource for supporting improved predictive toxicology. | |
Retrosynthesis Analysis
AI-Powered Synthesis Planning: Our tool employs the Template_relevance Pistachio, Template_relevance Bkms_metabolic, Template_relevance Pistachio_ringbreaker, Template_relevance Reaxys, Template_relevance Reaxys_biocatalysis model, leveraging a vast database of chemical reactions to predict feasible synthetic routes.
One-Step Synthesis Focus: Specifically designed for one-step synthesis, it provides concise and direct routes for your target compounds, streamlining the synthesis process.
Accurate Predictions: Utilizing the extensive PISTACHIO, BKMS_METABOLIC, PISTACHIO_RINGBREAKER, REAXYS, REAXYS_BIOCATALYSIS database, our tool offers high-accuracy predictions, reflecting the latest in chemical research and data.
Strategy Settings
Precursor scoring | Relevance Heuristic |
---|---|
Min. plausibility | 0.01 |
Model | Template_relevance |
Template Set | Pistachio/Bkms_metabolic/Pistachio_ringbreaker/Reaxys/Reaxys_biocatalysis |
Top-N result to add to graph | 6 |
Feasible Synthetic Routes
Disclaimer and Information on In-Vitro Research Products
Please be aware that all articles and product information presented on BenchChem are intended solely for informational purposes. The products available for purchase on BenchChem are specifically designed for in-vitro studies, which are conducted outside of living organisms. In-vitro studies, derived from the Latin term "in glass," involve experiments performed in controlled laboratory settings using cells or tissues. It is important to note that these products are not categorized as medicines or drugs, and they have not received approval from the FDA for the prevention, treatment, or cure of any medical condition, ailment, or disease. We must emphasize that any form of bodily introduction of these products into humans or animals is strictly prohibited by law. It is essential to adhere to these guidelines to ensure compliance with legal and ethical standards in research and experimentation.