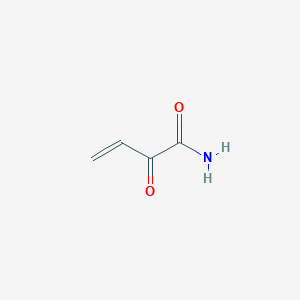
2-Oxobut-3-enamide
- Click on QUICK INQUIRY to receive a quote from our team of experts.
- With the quality product at a COMPETITIVE price, you can focus more on your research.
Overview
Description
2-Oxobut-3-enamide: (CAS number: 138616-23-6) is a compound with the chemical formula C₄H₅NO₂. . This molecule features a carbonyl group (C=O) and an unsaturated double bond (C=C) in its structure.
Preparation Methods
Synthetic Routes::
- One synthetic route involves the reaction of 5-(4-methoxyphenyl)furan-2,3-dione with urea in a 1:1 ratio, refluxed in a mixture of 1,2-dichloroethane-DMSO. This method yields (Z)-N-carbamoyl-4-hydroxy-4-(4-methoxyphenyl)-2-oxobut-3-enamide .
- Other methods may exist, but this is a notable example.
- Information on large-scale industrial production methods for 2-oxobut-3-enamide is limited. It is primarily synthesized in research laboratories.
Chemical Reactions Analysis
2-Oxobut-3-enamide: can undergo various reactions:
Scientific Research Applications
Medicinal Chemistry Applications
2-Oxobut-3-enamide derivatives have shown promise as bioactive compounds with various therapeutic potentials. Their structural characteristics allow them to act as intermediates in the synthesis of more complex molecules that exhibit biological activity.
Anticancer Activity
Recent studies have highlighted the anticancer properties of certain this compound derivatives. For instance, compounds derived from this structure have been evaluated for their cytotoxic effects on cancer cell lines, demonstrating significant inhibition of cell proliferation.
Case Study:
A study reported the synthesis of (Z)-N-Carbamoyl-4-hydroxy-4-(4-methoxyphenyl)-2-oxobut-3-enamide, which showed promising results against HepG2 liver cancer cells with an IC50 value of 2.57 µM. This indicates a strong potential for further development into therapeutic agents targeting specific cancer types .
Organic Synthesis Applications
The reactivity of this compound allows it to participate in various organic reactions, making it a valuable building block in synthetic chemistry.
Synthesis of α-Keto Amides
One notable application involves its use in the efficient synthesis of α-keto amides through condensation reactions with amines. This process allows for the formation of complex structures that are essential in pharmaceutical development.
Data Table: Synthesis of α-Keto Amides
Amine Type | Reaction Conditions | Yield (%) |
---|---|---|
Primary Amine | 0 °C, overnight stirring | 90 |
Secondary Amine | Room temperature, overnight stirring | 85 |
Cyclic Amine | 0 °C, overnight stirring | 88 |
This table summarizes the yields obtained from different amine types reacting with this compound under varying conditions .
Peptidic Coupling
Another significant application is in peptidic coupling reactions where this compound serves as a precursor for synthesizing peptide bonds. This method allows for the rapid assembly of peptide chains, which are crucial in drug development.
Case Study:
The reaction of β-γ-unsaturated α-keto acids with amines using BOPCl as a coupling agent resulted in high yields of β-γ-unsaturated α-keto amides. The methodology demonstrated versatility and efficiency in constructing peptide frameworks .
Mechanism of Action
- The exact mechanism by which 2-oxobut-3-enamide exerts its effects remains unclear. Further research is needed to elucidate its molecular targets and pathways.
Comparison with Similar Compounds
Similar Compounds:
Biological Activity
2-Oxobut-3-enamide, also referred to as α-ketoamide, is a compound of increasing interest in the field of medicinal chemistry due to its diverse biological activities. This article explores its biological activity, focusing on its mechanisms of action, cytotoxicity, anti-inflammatory properties, and potential therapeutic applications.
Chemical Structure and Properties
This compound is characterized by the presence of a carbonyl group adjacent to an amide functional group. This structural motif is significant for its interaction with biological targets.
The biological activity of this compound is primarily attributed to its ability to modulate enzyme activity and interact with various molecular pathways. For instance, studies have shown that α-ketoamides can inhibit enzymes such as phospholipase A2 (PLA2), which plays a crucial role in lipid metabolism and inflammatory processes .
Table 1: Enzyme Inhibition by this compound Derivatives
Cytotoxicity Studies
Cytotoxicity assays are essential for evaluating the safety profile of new compounds. The MTT assay is commonly used to assess cell viability in response to treatment with this compound. Results indicate that this compound exhibits varying degrees of cytotoxicity across different cell lines.
Table 2: Cytotoxicity of this compound on Various Cell Lines
Anti-inflammatory Activity
The anti-inflammatory properties of this compound have been investigated through various in vitro models. The compound has been shown to reduce the production of pro-inflammatory cytokines and inhibit the activation of nuclear factor kappa B (NF-κB), a key regulator of inflammation .
Case Study: Anti-inflammatory Effects
In a study examining the effects of α-ketoamides on inflammation, treatment with this compound resulted in a significant decrease in interleukin-6 (IL-6) levels in lipopolysaccharide-stimulated macrophages. This suggests potential therapeutic applications for conditions characterized by chronic inflammation.
Therapeutic Applications
Given its biological activities, this compound has potential applications in various therapeutic areas:
- Cancer Treatment : Due to its cytotoxic effects on cancer cell lines, there is potential for development as an anti-cancer agent.
- Anti-inflammatory Drugs : Its ability to modulate inflammatory pathways positions it as a candidate for treating inflammatory diseases.
- Metabolic Disorders : The inhibition of lipid metabolism enzymes suggests potential use in managing metabolic syndromes.
Properties
CAS No. |
138616-23-6 |
---|---|
Molecular Formula |
C4H5NO2 |
Molecular Weight |
99.09 g/mol |
IUPAC Name |
2-oxobut-3-enamide |
InChI |
InChI=1S/C4H5NO2/c1-2-3(6)4(5)7/h2H,1H2,(H2,5,7) |
InChI Key |
XYDCCJFDZNZRJD-UHFFFAOYSA-N |
Canonical SMILES |
C=CC(=O)C(=O)N |
Origin of Product |
United States |
Disclaimer and Information on In-Vitro Research Products
Please be aware that all articles and product information presented on BenchChem are intended solely for informational purposes. The products available for purchase on BenchChem are specifically designed for in-vitro studies, which are conducted outside of living organisms. In-vitro studies, derived from the Latin term "in glass," involve experiments performed in controlled laboratory settings using cells or tissues. It is important to note that these products are not categorized as medicines or drugs, and they have not received approval from the FDA for the prevention, treatment, or cure of any medical condition, ailment, or disease. We must emphasize that any form of bodily introduction of these products into humans or animals is strictly prohibited by law. It is essential to adhere to these guidelines to ensure compliance with legal and ethical standards in research and experimentation.