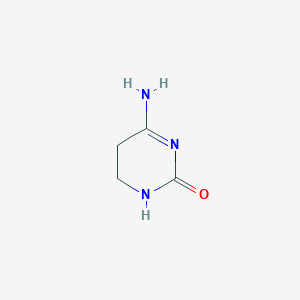
5,6-Dihydrocytosine
Overview
Description
5,6-Dihydrocytosine (DHC) is a saturated pyrimidine derivative characterized by the reduction of the 5,6-double bond in cytosine (Fig. 1). This structural modification imparts distinct chemical and biological properties, including enhanced susceptibility to hydrolytic deamination and tautomeric flexibility. DHC exists in equilibrium between amino (NH₂) and imino (NH) tautomeric forms, depending on the solvent polarity. In aqueous environments, the amino tautomer dominates, while non-polar solvents like chloroform favor the imino form .
DHC is biologically relevant as an intermediate in DNA repair and methylation processes. For instance, it forms transiently during the catalytic cycle of DNA methyltransferases (DNMTs), where a covalent this compound-DNA adduct is generated before methylation .
Preparation Methods
Synthetic Routes and Reaction Conditions
The synthesis of 5,6-Dihydrocytosine typically involves the hydrogenation of cytosine. This process can be carried out using a hydrogenation catalyst such as palladium on carbon (Pd/C) under a hydrogen atmosphere. The reaction conditions often include a solvent like ethanol or methanol and are conducted at room temperature or slightly elevated temperatures to ensure complete hydrogenation.
Industrial Production Methods
While specific industrial production methods for this compound are not well-documented, the general approach would involve scaling up the laboratory hydrogenation process. This would include optimizing the reaction conditions to maximize yield and purity, as well as implementing purification steps such as recrystallization or chromatography to isolate the desired product.
Chemical Reactions Analysis
Types of Reactions
5,6-Dihydrocytosine can undergo various chemical reactions, including:
Oxidation: This reaction can convert this compound into 5,6-dihydrouracil.
Reduction: Further reduction can lead to the formation of more saturated derivatives.
Substitution: Electrophilic substitution reactions can occur at the C-5 position, leading to products such as 5-iodo-5,6-dihydrocytosine.
Common Reagents and Conditions
Oxidation: Common oxidizing agents include hydrogen peroxide (H₂O₂) and potassium permanganate (KMnO₄).
Reduction: Catalysts like Pd/C are used under hydrogen gas.
Substitution: Reagents such as iodine (I₂) for iodination reactions.
Major Products
Oxidation: 5,6-Dihydrouracil.
Reduction: More saturated cytosine derivatives.
Substitution: 5-iodo-5,6-dihydrocytosine.
Scientific Research Applications
DNA Repair Mechanisms
5,6-Dihydrocytosine plays a significant role in the study of DNA damage and repair mechanisms. Research indicates that this compound can be formed during oxidative stress conditions affecting DNA. For instance, it can arise from the oxidation of cytosine, leading to the generation of unstable DNA lesions that may contribute to mutagenesis. Specifically, 5,6-dihydroxy-5,6-dihydrocytosine has been identified as a source of C → T transitions in DNA, which are critical for understanding mutation processes in organisms like E. coli .
Case Study: Oxidation of Cytosine
- Objective : To investigate how oxidized derivatives of cytosine contribute to mutations.
- Methodology : The oxidation of dCTP was performed using Fe²⁺ and H₂O₂, followed by HPLC isolation.
- Findings : The incorporation of oxidized species into viral genomes demonstrated high mutagenicity, highlighting the importance of this compound in genetic stability .
Photochemistry and Environmental Science
The compound has also been studied within the context of environmental science, particularly regarding its formation through photohydration reactions. Research has shown that ultraviolet (UV) irradiation can lead to the formation of this compound as a byproduct when treating emerging micropollutants . This application is particularly relevant for developing advanced oxidation processes aimed at degrading harmful pollutants in water.
Case Study: UV-Based Oxidation Processes
- Objective : To evaluate the effectiveness of UV irradiation on micropollutants.
- Methodology : The study involved analyzing the degradation products formed during UV treatment.
- Findings : The formation of this compound was noted as part of the degradation pathway for aromatic micropollutants, suggesting potential applications in wastewater treatment .
Chemical Reactivity and Deamination Studies
The reactivity of this compound has been extensively studied through computational methods. Detailed density functional theory (DFT) calculations have elucidated its deamination pathways in protic media. Two distinct pathways were identified: one occurring at acidic pH and another at neutral pH . Understanding these pathways is crucial for applications in biochemical research where deamination reactions are relevant.
Case Study: Mechanism Investigation
- Objective : To explore the deamination mechanisms of this compound.
- Methodology : DFT calculations were performed to model the reaction pathways.
- Findings : The study revealed that the nucleophilic addition is rate-determining with lower activation energies compared to unsaturated cytosine derivatives, indicating higher reactivity .
Synthesis and Analytical Applications
The synthesis of this compound derivatives is also significant for developing new biochemical tools. For example, its derivatives have been utilized as potent inhibitors for enzymes like cytosine-5-methyltransferases. This application is vital for epigenetic studies where modulation of methylation patterns can influence gene expression .
Synthesis Case Study
- Objective : To develop inhibitors for methyltransferases.
- Methodology : Synthesis of 5-methyl-6-Cys-81-S-5,6-dihydrocytosine was conducted using quantum mechanical/molecular mechanical methods.
- Findings : The synthesized compounds exhibited enhanced inhibition compared to traditional inhibitors like 5-fluoro-cytosine .
Mechanism of Action
The primary mechanism of action for 5,6-Dihydrocytosine involves its deamination to form 5,6-dihydrouracil. This reaction is facilitated by the nucleophilic addition of water to the C-4 position, followed by the elimination of an ammonium ion. The increased electrophilicity of the C-4 carbon in the dihydro form accelerates this process compared to unsaturated cytosine derivatives.
Comparison with Similar Compounds
Comparison with Structurally Similar Compounds
5,6-Dihydrocytosine vs. Cytosine
Key Findings :
- DHC deaminates ~10⁶-fold faster than cytosine due to reduced aromatic stability, forming uracil derivatives .
- Unlike cytosine, DHC participates in covalent catalysis with DNMTs, enabling methylation at the C5 position .
This compound vs. 5,6-Dihydrouracil
Key Findings :
- DHC’s amino group enables interactions with repair enzymes like SMUG1 and UNG, whereas dihydrouracil is processed by distinct glycosylases .
This compound vs. 5-Methylcytosine
Key Findings :
- 5-Methylcytosine is a stable epigenetic regulator, while DHC is a transient intermediate in its biosynthesis .
- DNMTs form a covalent bond with DHC during catalysis, a step absent in 5-methylcytosine processing .
This compound vs. Cytosine Glycol
Key Findings :
- Cytosine glycol is a bulky oxidation product repaired via base excision, whereas DHC is a non-bulky lesion excised via deamination-coupled repair .
Research Findings and Implications
- Deamination Dynamics : DHC’s rapid deamination (t₁/₂ ≈ 2 h) under physiological conditions contributes to C→T mutations in oxidative stress or UV-damaged DNA .
- Methylation Catalysis : DNMTs transiently stabilize DHC as a covalent intermediate, a mechanism exploited by inhibitors like 5-azacytosine .
- Tautomer-Dependent Repair: The imino tautomer of DHC in hydrophobic enzyme active sites may influence mismatch repair efficiency .
Biological Activity
5,6-Dihydrocytosine is a reduced form of cytosine that plays a significant role in various biological processes, including DNA repair mechanisms and the inhibition of DNA methyltransferases. This compound is particularly noteworthy due to its involvement in mutagenesis and its potential therapeutic applications.
-
DNA Repair and Mutagenesis :
- This compound is formed as an intermediate during the oxidation of cytosine. It participates in DNA repair processes by being excised from DNA strands by enzymes such as endonuclease III. Studies have shown that the half-life of cytosine glycols (which includes this compound) in double-stranded DNA is significantly extended, suggesting its importance in maintaining genomic integrity .
- The excision kinetics reveal that this compound is preferentially removed compared to other oxidized forms like 5-hydroxycytosine, indicating its role in mutagenesis .
-
Inhibition of DNA Methyltransferases :
- Research indicates that 5-aza-5,6-dihydrocytosine serves as a potent inhibitor of cytosine-5-methyltransferases. This enzyme family is crucial for DNA methylation processes, which are often dysregulated in cancer . The compound acts as a transition-state mimic, effectively binding to the active site of these enzymes and preventing their normal function .
Table 1: Summary of Biological Activities
Research Findings
- A study demonstrated that sodium bisulfite treatment leads to the formation of this compound-6-sulfonate as an intermediate during deamination reactions. This process highlights the compound's role in mutagenesis and its potential applications in genetic studies .
- Another investigation into the chemical reactivity of this compound revealed its capacity to induce lethal mutagenesis in viral systems, particularly HIV. This suggests a potential therapeutic use in antiviral strategies .
Q & A
Basic Research Questions
Q. What are the established methods for synthesizing and characterizing 5,6-dihydrocytosine in laboratory settings?
- Methodological Answer : this compound derivatives can be synthesized via enzymatic pathways involving methyltransferases, where cytosine reacts with formaldehyde to form a covalent intermediate (e.g., 5-hydroxymethylcytosine) . UV spectral analysis (e.g., 250–225 nm absorbance profiles) and comparative studies with sulfonate derivatives provide structural validation . Early synthesis protocols involve bacterial pyrimidine biosynthesis pathways, as demonstrated by Green & Cohen (1957) using radiolabeled precursors and chromatography for isolation .
Q. How can researchers detect and quantify cytosine deamination products, including this compound, in DNA?
- Methodological Answer : Sensitive genetic assays, such as those using E. coli mismatch repair systems, can detect deamination-induced mutations. Frederico et al. (1990) quantified deamination rates via kinetic studies under varying pH and temperature conditions, measuring activation energy (Eₐ) through Arrhenius plots . Theoretical models (e.g., density functional theory) predict hydrolytic deamination pathways, validated by NMR and mass spectrometry .
Advanced Research Questions
Q. What role does this compound play in enzymatic DNA methylation mechanisms?
- Methodological Answer : DNA cytosine-5 methyltransferases (C5-MTases) form a covalent this compound intermediate during methylation. This involves an SN2 reaction with S-adenosylmethionine (SAM) as a cofactor. Researchers can track this intermediate using radiolabeled SAM and gel electrophoresis to isolate methylated DNA products. Reversible formaldehyde cross-linking experiments further validate its transient role .
Q. How do computational studies explain the reactivity differences between cytosine, 5-methylcytosine, and this compound?
- Methodological Answer : Density functional theory (DFT) calculations reveal distinct reactivity indices at the C4 position. For this compound, reduced aromaticity increases susceptibility to nucleophilic attacks. Comparative electron density maps (∆f(⃗r)) and Fukui function analyses highlight electrophilic regions, guiding experimental design for selective derivatization .
Q. What mechanistic insights explain the mutagenic effects of this compound in DNA replication?
- Methodological Answer : this compound disrupts replication fidelity by altering tautomeric equilibria (K values shift from 10⁻⁴ to 1). In vitro transcription and translation assays with T7 RNA polymerase and E. coli ribosomes demonstrate misincorporation of adenine instead of guanine. Hydroxylamine mutagenesis parallels these effects, confirming tautomer-driven errors .
Q. How does this compound stability vary under radiolytic or photolytic conditions?
- Methodological Answer : Radiolysis (γ-irradiation) at pH 3–7 generates photohydrated cytosine derivatives, detectable via UV hypochromicity shifts at 250 nm. Stability assays under oxidative stress (e.g., H₂O₂ exposure) coupled with HPLC analysis quantify degradation products like cytosine glycol. These studies inform DNA damage repair models .
Q. What experimental strategies resolve contradictions in the regioselectivity of this compound derivatives in Diels-Alder reactions?
- Methodological Answer : Competitive cycloaddition experiments with 2-substituted furans (e.g., 2-tert-butylfuran) and DFT-optimized transition-state models explain regioselectivity. NMR (COSY, NOESY) and X-ray crystallography differentiate endo vs. exo adducts. Steric and electronic parameters (Hammett σ values) correlate with product ratios .
Q. Data Contradiction Analysis
Q. Why do some studies report this compound as a stable intermediate, while others classify it as a transient species?
- Methodological Answer : Stability depends on reaction milieu. In protic solvents, hydrolytic deamination dominates, making isolation challenging without rapid quenching (e.g., flash-freezing). Contrastingly, methyltransferase-bound intermediates are stabilized by enzyme active sites, enabling detection via stopped-flow kinetics .
Properties
IUPAC Name |
4-amino-5,6-dihydro-1H-pyrimidin-2-one | |
---|---|---|
Details | Computed by LexiChem 2.6.6 (PubChem release 2019.06.18) | |
Source | PubChem | |
URL | https://pubchem.ncbi.nlm.nih.gov | |
Description | Data deposited in or computed by PubChem | |
InChI |
InChI=1S/C4H7N3O/c5-3-1-2-6-4(8)7-3/h1-2H2,(H3,5,6,7,8) | |
Details | Computed by InChI 1.0.5 (PubChem release 2019.06.18) | |
Source | PubChem | |
URL | https://pubchem.ncbi.nlm.nih.gov | |
Description | Data deposited in or computed by PubChem | |
InChI Key |
FPOVCZDHZSAAIX-UHFFFAOYSA-N | |
Details | Computed by InChI 1.0.5 (PubChem release 2019.06.18) | |
Source | PubChem | |
URL | https://pubchem.ncbi.nlm.nih.gov | |
Description | Data deposited in or computed by PubChem | |
Canonical SMILES |
C1CNC(=O)N=C1N | |
Details | Computed by OEChem 2.1.5 (PubChem release 2019.06.18) | |
Source | PubChem | |
URL | https://pubchem.ncbi.nlm.nih.gov | |
Description | Data deposited in or computed by PubChem | |
Molecular Formula |
C4H7N3O | |
Details | Computed by PubChem 2.1 (PubChem release 2019.06.18) | |
Source | PubChem | |
URL | https://pubchem.ncbi.nlm.nih.gov | |
Description | Data deposited in or computed by PubChem | |
DSSTOX Substance ID |
DTXSID40978835 | |
Record name | 6-Imino-1,4,5,6-tetrahydropyrimidin-2-ol | |
Source | EPA DSSTox | |
URL | https://comptox.epa.gov/dashboard/DTXSID40978835 | |
Description | DSSTox provides a high quality public chemistry resource for supporting improved predictive toxicology. | |
Molecular Weight |
113.12 g/mol | |
Details | Computed by PubChem 2.1 (PubChem release 2021.05.07) | |
Source | PubChem | |
URL | https://pubchem.ncbi.nlm.nih.gov | |
Description | Data deposited in or computed by PubChem | |
CAS No. |
6297-70-7 | |
Record name | NSC46091 | |
Source | DTP/NCI | |
URL | https://dtp.cancer.gov/dtpstandard/servlet/dwindex?searchtype=NSC&outputformat=html&searchlist=46091 | |
Description | The NCI Development Therapeutics Program (DTP) provides services and resources to the academic and private-sector research communities worldwide to facilitate the discovery and development of new cancer therapeutic agents. | |
Explanation | Unless otherwise indicated, all text within NCI products is free of copyright and may be reused without our permission. Credit the National Cancer Institute as the source. | |
Record name | 6-Imino-1,4,5,6-tetrahydropyrimidin-2-ol | |
Source | EPA DSSTox | |
URL | https://comptox.epa.gov/dashboard/DTXSID40978835 | |
Description | DSSTox provides a high quality public chemistry resource for supporting improved predictive toxicology. | |
Disclaimer and Information on In-Vitro Research Products
Please be aware that all articles and product information presented on BenchChem are intended solely for informational purposes. The products available for purchase on BenchChem are specifically designed for in-vitro studies, which are conducted outside of living organisms. In-vitro studies, derived from the Latin term "in glass," involve experiments performed in controlled laboratory settings using cells or tissues. It is important to note that these products are not categorized as medicines or drugs, and they have not received approval from the FDA for the prevention, treatment, or cure of any medical condition, ailment, or disease. We must emphasize that any form of bodily introduction of these products into humans or animals is strictly prohibited by law. It is essential to adhere to these guidelines to ensure compliance with legal and ethical standards in research and experimentation.