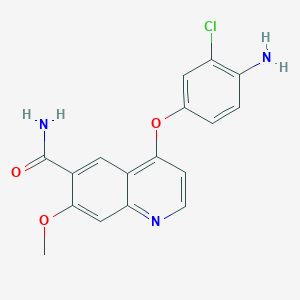
4-(4-Amino-3-chlorophenoxy)-7-methoxyquinoline-6-carboxamide
Overview
Description
4-(4-Amino-3-chlorophenoxy)-7-methoxyquinoline-6-carboxamide is a synthetic organic compound with a complex structure. It is related to Lenvatinib, an orally active inhibitor of multiple receptor tyrosine kinases, including vascular endothelial growth factor receptors, fibroblast growth factor receptors, and stem cell factor receptors . This compound is of significant interest in medicinal chemistry due to its potential therapeutic applications.
Mechanism of Action
Target of Action
The compound 4-(4-Amino-3-chlorophenoxy)-7-methoxyquinoline-6-carboxamide is related to Lenvatinib , an orally active inhibitor of multiple receptor tyrosine kinases . The primary targets of this compound are likely to be similar to those of Lenvatinib, which include VEGF (Vascular Endothelial Growth Factor), FGF (Fibroblast Growth Factor), and SCF (Stem Cell Factor) receptors . These receptors play crucial roles in angiogenesis and cell proliferation.
Biochemical Analysis
Biochemical Properties
4-(4-Amino-3-chlorophenoxy)-7-methoxyquinoline-6-carboxamide plays a significant role in biochemical reactions by interacting with various enzymes, proteins, and other biomolecules. It has been shown to inhibit multiple receptor tyrosine kinases, including vascular endothelial growth factor (VEGF) receptors, fibroblast growth factor (FGF) receptors, and stem cell factor (SCF) receptors . These interactions are crucial for its anti-angiogenic activity, which makes it a promising candidate for cancer therapy.
Cellular Effects
This compound exerts various effects on different cell types and cellular processes. It influences cell function by modulating cell signaling pathways, gene expression, and cellular metabolism. For instance, it has been observed to inhibit the proliferation of cancer cells by blocking the VEGF signaling pathway, leading to reduced angiogenesis and tumor growth . Additionally, it affects gene expression by downregulating the expression of pro-angiogenic factors and upregulating the expression of anti-angiogenic factors.
Molecular Mechanism
The molecular mechanism of this compound involves its binding interactions with receptor tyrosine kinases. By binding to the ATP-binding site of these receptors, it inhibits their kinase activity, preventing the phosphorylation of downstream signaling molecules . This inhibition disrupts key signaling pathways involved in cell proliferation, migration, and survival, ultimately leading to the suppression of angiogenesis and tumor growth.
Temporal Effects in Laboratory Settings
In laboratory settings, the effects of this compound have been studied over time to assess its stability, degradation, and long-term impact on cellular function. The compound has demonstrated good stability under standard laboratory conditions, with minimal degradation over extended periods . Long-term studies have shown that continuous exposure to the compound can lead to sustained inhibition of angiogenesis and tumor growth, indicating its potential for long-term therapeutic use.
Dosage Effects in Animal Models
The effects of this compound vary with different dosages in animal models. At lower doses, the compound effectively inhibits angiogenesis and tumor growth without causing significant toxicity . At higher doses, some toxic effects have been observed, including hepatotoxicity and nephrotoxicity. These findings highlight the importance of optimizing the dosage to achieve the desired therapeutic effects while minimizing adverse effects.
Metabolic Pathways
This compound is involved in various metabolic pathways, interacting with enzymes and cofactors that modulate its activity and metabolism. The compound is primarily metabolized by cytochrome P450 enzymes, which facilitate its biotransformation into active and inactive metabolites . These metabolic processes can influence the compound’s efficacy and toxicity, making it essential to understand its metabolic profile for effective therapeutic use.
Transport and Distribution
The transport and distribution of this compound within cells and tissues are mediated by specific transporters and binding proteins. The compound is known to interact with organic anion-transporting polypeptides (OATPs) and P-glycoprotein (P-gp), which facilitate its uptake and efflux across cellular membranes . These interactions can affect the compound’s localization, accumulation, and overall bioavailability, influencing its therapeutic potential.
Subcellular Localization
This compound exhibits specific subcellular localization patterns that impact its activity and function. The compound is predominantly localized in the cytoplasm, where it interacts with receptor tyrosine kinases and other signaling molecules . Additionally, post-translational modifications, such as phosphorylation, can influence its subcellular distribution and targeting to specific compartments or organelles, further modulating its biological effects.
Preparation Methods
Synthetic Routes and Reaction Conditions
The synthesis of 4-(4-Amino-3-chlorophenoxy)-7-methoxyquinoline-6-carboxamide typically involves multiple steps. One common method includes the reaction of 4-amino-3-chlorophenol with 7-methoxyquinoline-6-carboxylic acid under specific conditions to form the desired compound . The reaction conditions often require controlled temperatures and the use of catalysts to ensure high yield and purity.
Industrial Production Methods
Industrial production of this compound may involve large-scale synthesis using optimized reaction conditions to maximize efficiency and minimize costs. This includes the use of automated reactors, continuous flow systems, and advanced purification techniques to ensure the compound meets pharmaceutical-grade standards.
Chemical Reactions Analysis
Types of Reactions
4-(4-Amino-3-chlorophenoxy)-7-methoxyquinoline-6-carboxamide can undergo various chemical reactions, including:
Oxidation: This reaction involves the addition of oxygen or the removal of hydrogen, often using oxidizing agents like hydrogen peroxide or potassium permanganate.
Reduction: This reaction involves the addition of hydrogen or the removal of oxygen, typically using reducing agents like sodium borohydride or lithium aluminum hydride.
Substitution: This reaction involves the replacement of one functional group with another, often using reagents like halogens or nucleophiles.
Common Reagents and Conditions
Oxidation: Common oxidizing agents include hydrogen peroxide and potassium permanganate, often used under acidic or basic conditions.
Reduction: Common reducing agents include sodium borohydride and lithium aluminum hydride, typically used in anhydrous solvents.
Substitution: Common reagents include halogens (e.g., chlorine, bromine) and nucleophiles (e.g., amines, thiols), often used under controlled temperatures and inert atmospheres.
Major Products Formed
The major products formed from these reactions depend on the specific conditions and reagents used. For example, oxidation may yield quinoline derivatives, while reduction may produce amine derivatives. Substitution reactions can result in various substituted quinoline compounds.
Scientific Research Applications
4-(4-Amino-3-chlorophenoxy)-7-methoxyquinoline-6-carboxamide has several scientific research applications, including:
Chemistry: Used as a building block in the synthesis of more complex organic molecules.
Biology: Studied for its potential effects on cellular pathways and mechanisms.
Industry: Utilized in the development of pharmaceuticals and other chemical products.
Comparison with Similar Compounds
Similar Compounds
Sorafenib: Another multi-kinase inhibitor used in cancer treatment, targeting similar pathways.
Sunitinib: A multi-kinase inhibitor with applications in cancer therapy, targeting similar receptors.
Uniqueness
4-(4-Amino-3-chlorophenoxy)-7-methoxyquinoline-6-carboxamide is unique due to its specific structure and the combination of functional groups that allow it to interact with multiple receptor tyrosine kinases. This multi-target approach can enhance its therapeutic potential and reduce the likelihood of resistance development compared to single-target inhibitors.
Properties
IUPAC Name |
4-(4-amino-3-chlorophenoxy)-7-methoxyquinoline-6-carboxamide | |
---|---|---|
Source | PubChem | |
URL | https://pubchem.ncbi.nlm.nih.gov | |
Description | Data deposited in or computed by PubChem | |
InChI |
InChI=1S/C17H14ClN3O3/c1-23-16-8-14-10(7-11(16)17(20)22)15(4-5-21-14)24-9-2-3-13(19)12(18)6-9/h2-8H,19H2,1H3,(H2,20,22) | |
Source | PubChem | |
URL | https://pubchem.ncbi.nlm.nih.gov | |
Description | Data deposited in or computed by PubChem | |
InChI Key |
OFQLBCBNNWFEPV-UHFFFAOYSA-N | |
Source | PubChem | |
URL | https://pubchem.ncbi.nlm.nih.gov | |
Description | Data deposited in or computed by PubChem | |
Canonical SMILES |
COC1=CC2=NC=CC(=C2C=C1C(=O)N)OC3=CC(=C(C=C3)N)Cl | |
Source | PubChem | |
URL | https://pubchem.ncbi.nlm.nih.gov | |
Description | Data deposited in or computed by PubChem | |
Molecular Formula |
C17H14ClN3O3 | |
Source | PubChem | |
URL | https://pubchem.ncbi.nlm.nih.gov | |
Description | Data deposited in or computed by PubChem | |
Molecular Weight |
343.8 g/mol | |
Source | PubChem | |
URL | https://pubchem.ncbi.nlm.nih.gov | |
Description | Data deposited in or computed by PubChem | |
CAS No. |
417722-93-1 | |
Record name | 4-(4-Amino-3-chlorophenoxy)-7-methoxy-6-quinolinecarboxamide | |
Source | ChemIDplus | |
URL | https://pubchem.ncbi.nlm.nih.gov/substance/?source=chemidplus&sourceid=0417722931 | |
Description | ChemIDplus is a free, web search system that provides access to the structure and nomenclature authority files used for the identification of chemical substances cited in National Library of Medicine (NLM) databases, including the TOXNET system. | |
Record name | 4-(4-AMINO-3-CHLOROPHENOXY)-7-METHOXY-6-QUINOLINECARBOXAMIDE | |
Source | FDA Global Substance Registration System (GSRS) | |
URL | https://gsrs.ncats.nih.gov/ginas/app/beta/substances/ZTF97MBJ36 | |
Description | The FDA Global Substance Registration System (GSRS) enables the efficient and accurate exchange of information on what substances are in regulated products. Instead of relying on names, which vary across regulatory domains, countries, and regions, the GSRS knowledge base makes it possible for substances to be defined by standardized, scientific descriptions. | |
Explanation | Unless otherwise noted, the contents of the FDA website (www.fda.gov), both text and graphics, are not copyrighted. They are in the public domain and may be republished, reprinted and otherwise used freely by anyone without the need to obtain permission from FDA. Credit to the U.S. Food and Drug Administration as the source is appreciated but not required. | |
Retrosynthesis Analysis
AI-Powered Synthesis Planning: Our tool employs the Template_relevance Pistachio, Template_relevance Bkms_metabolic, Template_relevance Pistachio_ringbreaker, Template_relevance Reaxys, Template_relevance Reaxys_biocatalysis model, leveraging a vast database of chemical reactions to predict feasible synthetic routes.
One-Step Synthesis Focus: Specifically designed for one-step synthesis, it provides concise and direct routes for your target compounds, streamlining the synthesis process.
Accurate Predictions: Utilizing the extensive PISTACHIO, BKMS_METABOLIC, PISTACHIO_RINGBREAKER, REAXYS, REAXYS_BIOCATALYSIS database, our tool offers high-accuracy predictions, reflecting the latest in chemical research and data.
Strategy Settings
Precursor scoring | Relevance Heuristic |
---|---|
Min. plausibility | 0.01 |
Model | Template_relevance |
Template Set | Pistachio/Bkms_metabolic/Pistachio_ringbreaker/Reaxys/Reaxys_biocatalysis |
Top-N result to add to graph | 6 |
Feasible Synthetic Routes
Q1: Given the structural similarities between 4-(4-Amino-3-chlorophenoxy)-7-methoxyquinoline-6-carboxamide and Lenvatinib, could the novel synthesis method described in the paper be applicable or adaptable for synthesizing this compound?
A1: It's possible. The paper describes a new approach to synthesizing Lenvatinib using 4-nitrophenyl cyclopropylcarbamate. [] While we don't have data on the direct applicability to this compound, the shared structural motifs suggest that adaptations of this method might be explored for its synthesis. Further research would be necessary to determine the feasibility and efficiency of such adaptations.
Disclaimer and Information on In-Vitro Research Products
Please be aware that all articles and product information presented on BenchChem are intended solely for informational purposes. The products available for purchase on BenchChem are specifically designed for in-vitro studies, which are conducted outside of living organisms. In-vitro studies, derived from the Latin term "in glass," involve experiments performed in controlled laboratory settings using cells or tissues. It is important to note that these products are not categorized as medicines or drugs, and they have not received approval from the FDA for the prevention, treatment, or cure of any medical condition, ailment, or disease. We must emphasize that any form of bodily introduction of these products into humans or animals is strictly prohibited by law. It is essential to adhere to these guidelines to ensure compliance with legal and ethical standards in research and experimentation.