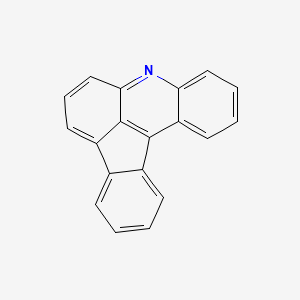
Indeno(1,2,3-kl)acridine
- Click on QUICK INQUIRY to receive a quote from our team of experts.
- With the quality product at a COMPETITIVE price, you can focus more on your research.
Overview
Description
Indeno(1,2,3-kl)acridine is a polycyclic aromatic compound that belongs to the class of acridine derivatives These compounds are known for their complex fused ring structures, which contribute to their unique chemical and physical properties
Preparation Methods
Synthetic Routes and Reaction Conditions: The synthesis of Indeno(1,2,3-kl)acridine typically involves multi-step organic reactions. One common method is the intramolecular Povarov reaction, which involves the cyclization of aromatic amines with aldehydes and olefins under acidic conditions . This reaction is known for its efficiency in constructing nitrogen-containing heterocycles.
Industrial Production Methods: Industrial production of this compound may involve the use of advanced catalytic systems to enhance yield and selectivity. For instance, the use of acidic magnetic dendrimers as catalysts has been reported to facilitate the synthesis of related compounds under mild conditions . These catalysts can be easily separated and recycled, making the process more sustainable.
Chemical Reactions Analysis
Types of Reactions: Indeno(1,2,3-kl)acridine undergoes various chemical reactions, including:
Oxidation: This reaction can be carried out using oxidizing agents such as potassium permanganate or chromium trioxide.
Reduction: Reduction reactions typically involve the use of reducing agents like lithium aluminum hydride or sodium borohydride.
Substitution: Electrophilic substitution reactions can occur at the aromatic rings, often using reagents like halogens or nitrating agents.
Common Reagents and Conditions:
Oxidation: Potassium permanganate in acidic medium.
Reduction: Lithium aluminum hydride in dry ether.
Substitution: Chlorine gas in the presence of a catalyst like iron(III) chloride.
Major Products: The major products formed from these reactions depend on the specific conditions and reagents used. For example, oxidation may yield quinones, while reduction can produce dihydro derivatives.
Scientific Research Applications
Mechanism of Action
The primary mechanism by which Indeno(1,2,3-kl)acridine exerts its effects is through DNA intercalation. This process involves the insertion of the compound between DNA base pairs, leading to the disruption of essential biological processes such as DNA replication and transcription . The compound’s planar structure allows it to fit snugly between the base pairs, stabilizing the DNA-intercalator complex and inhibiting the activity of DNA-dependent enzymes.
Comparison with Similar Compounds
- Benz[c]indeno[1,3-kl]acridine
- Phenanthro[9,10,1-mna]acridine
- Dibenz[c,h]indeno[1,3-kl]acridine
Uniqueness: Indeno(1,2,3-kl)acridine is unique due to its specific ring fusion pattern, which imparts distinct electronic and steric properties. This uniqueness makes it particularly valuable in applications requiring precise molecular interactions, such as DNA intercalation and organic electronics .
Biological Activity
Indeno(1,2,3-kl)acridine is a polycyclic aromatic hydrocarbon that has garnered attention for its potential biological activities, particularly in the context of cancer research. This compound belongs to the indenoacridine family, characterized by its unique structure that facilitates interactions with biological macromolecules such as DNA and proteins. This article explores the biological activity of this compound, focusing on its anticancer properties, mechanisms of action, and relevant research findings.
Chemical Structure and Properties
This compound has a molecular formula of C19H11N and a molecular weight of approximately 253.3 g/mol. Its structure consists of multiple fused aromatic rings which contribute to its electronic properties and biological interactions. The compound's ability to intercalate into DNA is a key feature that underlies its anticancer potential.
The primary mechanism through which this compound exerts its biological effects is through DNA intercalation . This process disrupts normal cellular functions such as replication and transcription, leading to cell death. The compound's interaction with DNA can result in the formation of DNA adducts, which are critical in understanding its cytotoxic effects.
Key Mechanisms:
- Intercalation into DNA : Disrupts replication and transcription.
- Formation of DNA Adducts : Alters genetic material leading to mutations.
- Inhibition of Enzymatic Activity : Affects various enzymes and proteins involved in cellular processes.
Anticancer Properties
This compound has been extensively studied for its anticancer properties. Research indicates that it exhibits significant cytotoxic effects against various cancer cell lines. The following table summarizes some key findings from recent studies:
Cell Line | IC50 (µM) | Effect Observed |
---|---|---|
HeLa (cervical cancer) | 5.0 | Induction of apoptosis |
MCF-7 (breast cancer) | 4.5 | Cell cycle arrest |
HCT-116 (colon cancer) | 3.8 | Inhibition of proliferation |
K562 (leukemia) | 6.0 | Increased DNA fragmentation |
These studies demonstrate that this compound can effectively inhibit the growth of various cancer cells through mechanisms such as apoptosis induction and cell cycle arrest.
Case Studies and Research Findings
-
Study on Antitumor Activity :
A study published in Cancer Letters evaluated the cytotoxicity of this compound against several human cancer cell lines. Results indicated that the compound significantly inhibited cell viability in a dose-dependent manner, with the most potent effects observed in HeLa cells . -
Mechanistic Insights :
Research published in Molecular Cancer Therapeutics explored the molecular mechanisms underlying the anticancer activity of this compound. The study found that treatment with the compound led to increased levels of phosphorylated p53 and activated caspases, indicating the induction of apoptosis through a mitochondrial pathway . -
Comparative Analysis :
A comparative study highlighted how structural modifications to indenoacridines could enhance their biological activity. It was noted that certain derivatives exhibited improved binding affinities for DNA and increased cytotoxicity compared to this compound itself .
Properties
CAS No. |
386-77-6 |
---|---|
Molecular Formula |
C19H11N |
Molecular Weight |
253.3 g/mol |
IUPAC Name |
13-azapentacyclo[10.7.1.02,7.08,20.014,19]icosa-1(20),2,4,6,8,10,12,14,16,18-decaene |
InChI |
InChI=1S/C19H11N/c1-2-7-13-12(6-1)14-9-5-11-17-19(14)18(13)15-8-3-4-10-16(15)20-17/h1-11H |
InChI Key |
QKNNKYUQAGCZNS-UHFFFAOYSA-N |
Canonical SMILES |
C1=CC=C2C(=C1)C3=CC=CC4=NC5=CC=CC=C5C2=C43 |
Origin of Product |
United States |
Disclaimer and Information on In-Vitro Research Products
Please be aware that all articles and product information presented on BenchChem are intended solely for informational purposes. The products available for purchase on BenchChem are specifically designed for in-vitro studies, which are conducted outside of living organisms. In-vitro studies, derived from the Latin term "in glass," involve experiments performed in controlled laboratory settings using cells or tissues. It is important to note that these products are not categorized as medicines or drugs, and they have not received approval from the FDA for the prevention, treatment, or cure of any medical condition, ailment, or disease. We must emphasize that any form of bodily introduction of these products into humans or animals is strictly prohibited by law. It is essential to adhere to these guidelines to ensure compliance with legal and ethical standards in research and experimentation.