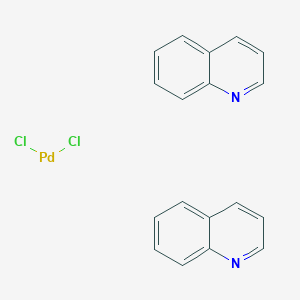
Palladium, dichlorobis(quinoline)-
- Click on QUICK INQUIRY to receive a quote from our team of experts.
- With the quality product at a COMPETITIVE price, you can focus more on your research.
Overview
Description
Palladium, dichlorobis(quinoline)-: is a coordination compound that features palladium as the central metal atom coordinated to two chloride ions and two quinoline ligands. This compound is of significant interest in the field of organometallic chemistry due to its versatile catalytic properties and its role in various chemical reactions, particularly in the synthesis of heterocyclic compounds.
Preparation Methods
Synthetic Routes and Reaction Conditions: The synthesis of palladium, dichlorobis(quinoline)- typically involves the reaction of palladium(II) chloride with quinoline in an appropriate solvent. The reaction is usually carried out under reflux conditions to ensure complete coordination of the quinoline ligands to the palladium center. The general reaction can be represented as follows:
[ \text{PdCl}_2 + 2 \text{C}_9\text{H}_7\text{N} \rightarrow \text{PdCl}_2(\text{C}_9\text{H}_7\text{N})_2 ]
Industrial Production Methods: In an industrial setting, the production of palladium, dichlorobis(quinoline)- may involve more scalable methods such as continuous flow synthesis. This approach allows for better control over reaction conditions and can lead to higher yields and purity of the final product.
Chemical Reactions Analysis
Types of Reactions: Palladium, dichlorobis(quinoline)- is known to undergo various types of chemical reactions, including:
Oxidation: The compound can participate in oxidation reactions where the palladium center is oxidized to a higher oxidation state.
Reduction: It can also be reduced back to palladium(0) under appropriate conditions.
Substitution: The chloride ligands can be substituted by other ligands such as phosphines or amines.
Common Reagents and Conditions:
Oxidation: Common oxidizing agents include oxygen, hydrogen peroxide, and other peroxides.
Reduction: Reducing agents such as hydrogen gas or hydrazine are often used.
Substitution: Ligand substitution reactions typically involve the use of excess ligand and may require heating or the presence of a base to facilitate the reaction.
Major Products: The major products formed from these reactions depend on the specific reagents and conditions used. For example, substitution of the chloride ligands with phosphines can lead to the formation of palladium-phosphine complexes, which are valuable catalysts in various organic transformations.
Scientific Research Applications
Chemistry: Palladium, dichlorobis(quinoline)- is widely used as a catalyst in organic synthesis, particularly in cross-coupling reactions such as the Suzuki-Miyaura and Heck reactions. These reactions are essential for forming carbon-carbon bonds in the synthesis of complex organic molecules.
Biology and Medicine: In biological and medicinal research, palladium complexes, including palladium, dichlorobis(quinoline)-, are investigated for their potential anticancer properties. The compound’s ability to interact with DNA and proteins makes it a candidate for developing new therapeutic agents.
Industry: In the industrial sector, palladium, dichlorobis(quinoline)- is used in the production of fine chemicals and pharmaceuticals. Its catalytic properties are harnessed to improve the efficiency and selectivity of various chemical processes.
Mechanism of Action
The mechanism by which palladium, dichlorobis(quinoline)- exerts its effects is primarily through its role as a catalyst. The palladium center can undergo oxidative addition, reductive elimination, and ligand exchange processes, which are crucial steps in catalytic cycles. These processes enable the compound to facilitate a wide range of chemical transformations by lowering the activation energy and increasing the reaction rate.
Comparison with Similar Compounds
Palladium, dichlorobis(triphenylphosphine)-: This compound features triphenylphosphine ligands instead of quinoline and is also widely used as a catalyst in organic synthesis.
Palladium, dichlorobis(benzonitrile)-: Benzonitrile ligands replace quinoline in this compound, and it is used in similar catalytic applications.
Uniqueness: Palladium, dichlorobis(quinoline)- is unique due to the presence of quinoline ligands, which can influence the electronic properties and reactivity of the palladium center. The quinoline ligands can also participate in π-π interactions, potentially enhancing the compound’s stability and catalytic efficiency.
Properties
Molecular Formula |
C18H14Cl2N2Pd |
---|---|
Molecular Weight |
435.6 g/mol |
IUPAC Name |
dichloropalladium;quinoline |
InChI |
InChI=1S/2C9H7N.2ClH.Pd/c2*1-2-6-9-8(4-1)5-3-7-10-9;;;/h2*1-7H;2*1H;/q;;;;+2/p-2 |
InChI Key |
WNRIITWJLQUJOM-UHFFFAOYSA-L |
Canonical SMILES |
C1=CC=C2C(=C1)C=CC=N2.C1=CC=C2C(=C1)C=CC=N2.Cl[Pd]Cl |
Origin of Product |
United States |
Disclaimer and Information on In-Vitro Research Products
Please be aware that all articles and product information presented on BenchChem are intended solely for informational purposes. The products available for purchase on BenchChem are specifically designed for in-vitro studies, which are conducted outside of living organisms. In-vitro studies, derived from the Latin term "in glass," involve experiments performed in controlled laboratory settings using cells or tissues. It is important to note that these products are not categorized as medicines or drugs, and they have not received approval from the FDA for the prevention, treatment, or cure of any medical condition, ailment, or disease. We must emphasize that any form of bodily introduction of these products into humans or animals is strictly prohibited by law. It is essential to adhere to these guidelines to ensure compliance with legal and ethical standards in research and experimentation.