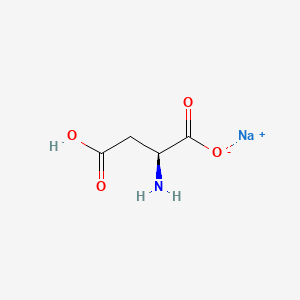
Monosodium aspartate
Overview
Description
Monosodium aspartate is the sodium salt of aspartic acid, a nonessential amino acid . It is used as an additive to cardioplegia solutions .
Synthesis Analysis
Monosodium aspartate can be produced through fermentation . The process involves the use of bacteria that secrete glutamic acid through their cell walls . The fermentation broth’s pH is adjusted to obtain L-glutamic acid crystals, which are then converted to monosodium aspartate .
Molecular Structure Analysis
Monosodium aspartate has the molecular formula C4H6NNaO4 . Its molecular weight is 155.08 g/mol . The structure of monosodium aspartate includes a sodium ion and an aspartate molecule .
Chemical Reactions Analysis
Monosodium aspartate, like other amino acids, can participate in various chemical reactions. For instance, it can be involved in transamination reactions with α-ketoglutarate, contributing to the biosynthesis of all amino acids .
Physical And Chemical Properties Analysis
Monosodium aspartate is a white crystalline powder that is significantly soluble in water . Its molecular weight is 155.08 g/mol, and it has a complex structure that includes sodium ions and aspartic acid .
Scientific Research Applications
Metabolic Engineering
Monosodium aspartate is used in metabolic engineering to enhance the production of L-aspartate and its derivatives. This involves the manipulation of metabolic pathways in microorganisms to optimize the production of compounds like β-alanine, ectoine, and D-pantothenic acid. The goal is to create cell factories that can sustainably produce these chemicals at an industrial scale .
Biochemical Studies
In biochemical research, monosodium aspartate serves as a model compound to study transport properties in cells. Its diffusion coefficients are measured to understand the movement of molecules through cellular membranes, which is crucial for drug delivery and controlled release applications .
Neurotransmitter Research
This compound is also significant in neuroscience as it acts as a principal neurotransmitter for fast synaptic excitation. Research on monosodium aspartate can lead to a better understanding of neurological processes and the development of treatments for various neurological disorders .
Food Science
In the field of food science, monosodium aspartate is explored for its role in flavor enhancement . It contributes to the umami taste and is used in the synthesis of aspartame, a widely used artificial sweetener .
Pharmaceutical Development
Monosodium aspartate is involved in the design and development of new pharmaceuticals . Its properties can influence the efficacy and delivery of drugs, making it a valuable compound in medicinal chemistry .
Material Science
Lastly, in material science, monosodium aspartate is used to develop advanced materials . It’s involved in the creation of polynucleotides for sensing and light-emitting devices, showcasing its versatility beyond biological applications .
Future Directions
properties
IUPAC Name |
sodium;(2S)-2-amino-4-hydroxy-4-oxobutanoate | |
---|---|---|
Source | PubChem | |
URL | https://pubchem.ncbi.nlm.nih.gov | |
Description | Data deposited in or computed by PubChem | |
InChI |
InChI=1S/C4H7NO4.Na/c5-2(4(8)9)1-3(6)7;/h2H,1,5H2,(H,6,7)(H,8,9);/q;+1/p-1/t2-;/m0./s1 | |
Source | PubChem | |
URL | https://pubchem.ncbi.nlm.nih.gov | |
Description | Data deposited in or computed by PubChem | |
InChI Key |
WTWSHHITWMVLBX-DKWTVANSSA-M | |
Source | PubChem | |
URL | https://pubchem.ncbi.nlm.nih.gov | |
Description | Data deposited in or computed by PubChem | |
Canonical SMILES |
C(C(C(=O)[O-])N)C(=O)O.[Na+] | |
Source | PubChem | |
URL | https://pubchem.ncbi.nlm.nih.gov | |
Description | Data deposited in or computed by PubChem | |
Isomeric SMILES |
C([C@@H](C(=O)[O-])N)C(=O)O.[Na+] | |
Source | PubChem | |
URL | https://pubchem.ncbi.nlm.nih.gov | |
Description | Data deposited in or computed by PubChem | |
Molecular Formula |
C4H6NNaO4 | |
Source | PubChem | |
URL | https://pubchem.ncbi.nlm.nih.gov | |
Description | Data deposited in or computed by PubChem | |
Related CAS |
56-84-8 (Parent) | |
Record name | Sodium aspartate | |
Source | ChemIDplus | |
URL | https://pubchem.ncbi.nlm.nih.gov/substance/?source=chemidplus&sourceid=0003792505 | |
Description | ChemIDplus is a free, web search system that provides access to the structure and nomenclature authority files used for the identification of chemical substances cited in National Library of Medicine (NLM) databases, including the TOXNET system. | |
DSSTOX Substance ID |
DTXSID9020904 | |
Record name | Monosodium aspartate | |
Source | EPA DSSTox | |
URL | https://comptox.epa.gov/dashboard/DTXSID9020904 | |
Description | DSSTox provides a high quality public chemistry resource for supporting improved predictive toxicology. | |
Molecular Weight |
155.08 g/mol | |
Source | PubChem | |
URL | https://pubchem.ncbi.nlm.nih.gov | |
Description | Data deposited in or computed by PubChem | |
Product Name |
Monosodium aspartate | |
CAS RN |
3792-50-5, 17090-93-6 | |
Record name | Sodium aspartate | |
Source | ChemIDplus | |
URL | https://pubchem.ncbi.nlm.nih.gov/substance/?source=chemidplus&sourceid=0003792505 | |
Description | ChemIDplus is a free, web search system that provides access to the structure and nomenclature authority files used for the identification of chemical substances cited in National Library of Medicine (NLM) databases, including the TOXNET system. | |
Record name | L-Aspartic acid, sodium salt (1:1) | |
Source | EPA Chemicals under the TSCA | |
URL | https://www.epa.gov/chemicals-under-tsca | |
Description | EPA Chemicals under the Toxic Substances Control Act (TSCA) collection contains information on chemicals and their regulations under TSCA, including non-confidential content from the TSCA Chemical Substance Inventory and Chemical Data Reporting. | |
Record name | Monosodium aspartate | |
Source | EPA DSSTox | |
URL | https://comptox.epa.gov/dashboard/DTXSID9020904 | |
Description | DSSTox provides a high quality public chemistry resource for supporting improved predictive toxicology. | |
Record name | Sodium hydrogen L-aspartate | |
Source | European Chemicals Agency (ECHA) | |
URL | https://echa.europa.eu/substance-information/-/substanceinfo/100.021.150 | |
Description | The European Chemicals Agency (ECHA) is an agency of the European Union which is the driving force among regulatory authorities in implementing the EU's groundbreaking chemicals legislation for the benefit of human health and the environment as well as for innovation and competitiveness. | |
Explanation | Use of the information, documents and data from the ECHA website is subject to the terms and conditions of this Legal Notice, and subject to other binding limitations provided for under applicable law, the information, documents and data made available on the ECHA website may be reproduced, distributed and/or used, totally or in part, for non-commercial purposes provided that ECHA is acknowledged as the source: "Source: European Chemicals Agency, http://echa.europa.eu/". Such acknowledgement must be included in each copy of the material. ECHA permits and encourages organisations and individuals to create links to the ECHA website under the following cumulative conditions: Links can only be made to webpages that provide a link to the Legal Notice page. | |
Record name | L-aspartic acid, sodium salt | |
Source | European Chemicals Agency (ECHA) | |
URL | https://echa.europa.eu/substance-information/-/substanceinfo/100.037.399 | |
Description | The European Chemicals Agency (ECHA) is an agency of the European Union which is the driving force among regulatory authorities in implementing the EU's groundbreaking chemicals legislation for the benefit of human health and the environment as well as for innovation and competitiveness. | |
Explanation | Use of the information, documents and data from the ECHA website is subject to the terms and conditions of this Legal Notice, and subject to other binding limitations provided for under applicable law, the information, documents and data made available on the ECHA website may be reproduced, distributed and/or used, totally or in part, for non-commercial purposes provided that ECHA is acknowledged as the source: "Source: European Chemicals Agency, http://echa.europa.eu/". Such acknowledgement must be included in each copy of the material. ECHA permits and encourages organisations and individuals to create links to the ECHA website under the following cumulative conditions: Links can only be made to webpages that provide a link to the Legal Notice page. | |
Retrosynthesis Analysis
AI-Powered Synthesis Planning: Our tool employs the Template_relevance Pistachio, Template_relevance Bkms_metabolic, Template_relevance Pistachio_ringbreaker, Template_relevance Reaxys, Template_relevance Reaxys_biocatalysis model, leveraging a vast database of chemical reactions to predict feasible synthetic routes.
One-Step Synthesis Focus: Specifically designed for one-step synthesis, it provides concise and direct routes for your target compounds, streamlining the synthesis process.
Accurate Predictions: Utilizing the extensive PISTACHIO, BKMS_METABOLIC, PISTACHIO_RINGBREAKER, REAXYS, REAXYS_BIOCATALYSIS database, our tool offers high-accuracy predictions, reflecting the latest in chemical research and data.
Strategy Settings
Precursor scoring | Relevance Heuristic |
---|---|
Min. plausibility | 0.01 |
Model | Template_relevance |
Template Set | Pistachio/Bkms_metabolic/Pistachio_ringbreaker/Reaxys/Reaxys_biocatalysis |
Top-N result to add to graph | 6 |
Feasible Synthetic Routes
Disclaimer and Information on In-Vitro Research Products
Please be aware that all articles and product information presented on BenchChem are intended solely for informational purposes. The products available for purchase on BenchChem are specifically designed for in-vitro studies, which are conducted outside of living organisms. In-vitro studies, derived from the Latin term "in glass," involve experiments performed in controlled laboratory settings using cells or tissues. It is important to note that these products are not categorized as medicines or drugs, and they have not received approval from the FDA for the prevention, treatment, or cure of any medical condition, ailment, or disease. We must emphasize that any form of bodily introduction of these products into humans or animals is strictly prohibited by law. It is essential to adhere to these guidelines to ensure compliance with legal and ethical standards in research and experimentation.