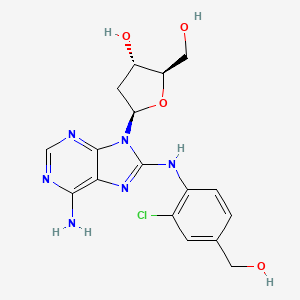
Adenosine, 8-((2-chloro-4-(hydroxymethyl)phenyl)amino)-2'-deoxy-
- Click on QUICK INQUIRY to receive a quote from our team of experts.
- With the quality product at a COMPETITIVE price, you can focus more on your research.
Overview
Description
(2R,3S,5R)-5-(6-Amino-8-((2-chloro-4-(hydroxymethyl)phenyl)amino)-9H-purin-9-yl)-2-(hydroxymethyl)tetrahydrofuran-3-ol is a complex organic compound with significant applications in various scientific fields. This compound is characterized by its unique structure, which includes a purine base linked to a tetrahydrofuran ring. It is often studied for its potential biological and medicinal properties.
Preparation Methods
Synthetic Routes and Reaction Conditions
The synthesis of (2R,3S,5R)-5-(6-Amino-8-((2-chloro-4-(hydroxymethyl)phenyl)amino)-9H-purin-9-yl)-2-(hydroxymethyl)tetrahydrofuran-3-ol typically involves multiple steps:
Formation of the Purine Base: The purine base is synthesized through a series of reactions starting from simple precursors like formamide and glycine.
Chlorination: The purine base is then chlorinated using reagents such as thionyl chloride or phosphorus oxychloride to introduce the chloro group.
Amination: The chlorinated purine is reacted with 2-chloro-4-(hydroxymethyl)aniline under controlled conditions to form the desired amino derivative.
Coupling with Tetrahydrofuran Ring: The final step involves coupling the amino-purine derivative with a tetrahydrofuran ring, which is achieved through a series of protection and deprotection steps to ensure the correct stereochemistry.
Industrial Production Methods
Industrial production of this compound may involve similar synthetic routes but on a larger scale. The process is optimized for yield and purity, often using automated reactors and continuous flow systems to maintain consistent reaction conditions. Purification steps such as crystallization, chromatography, and recrystallization are employed to obtain the final product with high purity.
Chemical Reactions Analysis
Types of Reactions
Oxidation: The hydroxymethyl groups in the compound can undergo oxidation to form aldehydes or carboxylic acids.
Reduction: The nitro groups, if present, can be reduced to amines using reducing agents like hydrogen gas in the presence of a catalyst.
Substitution: The chloro group can be substituted with other nucleophiles such as amines or thiols under appropriate conditions.
Common Reagents and Conditions
Oxidation: Potassium permanganate (KMnO4) or chromium trioxide (CrO3) in acidic medium.
Reduction: Hydrogen gas (H2) with palladium on carbon (Pd/C) as a catalyst.
Substitution: Sodium azide (NaN3) or thiourea in polar solvents like dimethyl sulfoxide (DMSO).
Major Products
Oxidation: Formation of aldehydes or carboxylic acids.
Reduction: Formation of primary or secondary amines.
Substitution: Formation of substituted purine derivatives.
Scientific Research Applications
Chemistry
Synthesis of Nucleoside Analogues: The compound is used as a precursor in the synthesis of various nucleoside analogues, which are important in medicinal chemistry.
Biology
Enzyme Inhibition Studies: It is used to study the inhibition of enzymes involved in nucleotide metabolism.
Medicine
Antiviral Agents: The compound and its derivatives are investigated for their potential as antiviral agents, particularly against retroviruses.
Industry
Pharmaceutical Manufacturing: It is used in the production of pharmaceutical intermediates and active pharmaceutical ingredients (APIs).
Mechanism of Action
The compound exerts its effects primarily through interaction with enzymes involved in nucleotide metabolism. It can act as a competitive inhibitor, binding to the active site of the enzyme and preventing the natural substrate from binding. This inhibition can disrupt the replication of viral genomes, making it a potential antiviral agent. The molecular targets include enzymes like reverse transcriptase and DNA polymerase.
Comparison with Similar Compounds
Similar Compounds
Acyclovir: A nucleoside analogue used as an antiviral agent.
Ganciclovir: Another antiviral nucleoside analogue with a similar mechanism of action.
Uniqueness
(2R,3S,5R)-5-(6-Amino-8-((2-chloro-4-(hydroxymethyl)phenyl)amino)-9H-purin-9-yl)-2-(hydroxymethyl)tetrahydrofuran-3-ol is unique due to its specific stereochemistry and the presence of both chloro and hydroxymethyl groups, which confer distinct chemical properties and biological activities compared to other nucleoside analogues.
Biological Activity
Adenosine, 8-((2-chloro-4-(hydroxymethyl)phenyl)amino)-2'-deoxy-, commonly referred to as compound 119631-05-9, is a modified adenosine derivative that has garnered attention for its potential biological activities, particularly in relation to adenosine receptors (ARs). This article explores its biological activity, focusing on its interactions with various adenosine receptors and its implications in therapeutic applications.
The compound's chemical structure is characterized by the presence of a chloro-substituted phenyl group at the 8-position of the adenosine molecule. The molecular formula is C17H19ClN6O4, and it has a molecular weight of approximately 388.82 g/mol .
Adenosine operates primarily through its interaction with four subtypes of adenosine receptors: A1, A2A, A2B, and A3. Each receptor subtype mediates different physiological responses:
- A1 Receptors : Activation typically results in decreased heart rate and neurotransmitter release.
- A2A Receptors : These are implicated in vasodilation and inhibition of platelet aggregation.
- A2B Receptors : They play a role in inflammatory responses.
- A3 Receptors : Involved in various cellular processes including apoptosis and immune response modulation.
Research indicates that the compound exhibits selective agonist activity at A1 and A2A receptors, which may contribute to its pharmacological effects .
In Vitro Studies
In vitro studies have demonstrated that adenosine derivatives can modulate intracellular signaling pathways through cAMP levels. For instance, activation of A2A receptors enhances cAMP production, leading to inhibition of platelet aggregation .
Table 1 summarizes the binding affinities (Ki values) for different adenosine receptor subtypes:
Receptor Type | Binding Affinity (Ki, nM) |
---|---|
A1 | 50 |
A2A | 180 |
A2B | 10 |
A3 | 200 |
In Vivo Studies
In vivo studies have shown that compounds similar to adenosine exhibit significant effects on physiological parameters such as heart rate and body temperature regulation. For example, certain derivatives have been reported to induce hypothermia via central A1 receptor activation without excessive peripheral effects .
Case Studies
- Anti-Platelet Activity : Research has demonstrated that compounds like 2-chloroadenosine (a structural analogue) inhibit platelet aggregation effectively at concentrations around 1.6 µM, showcasing the potential of similar derivatives in anti-thrombotic therapies .
- Neuroprotective Effects : Studies suggest that selective A1 receptor agonists can provide neuroprotective effects in models of ischemia and neurodegeneration, indicating a therapeutic avenue for conditions like stroke or Alzheimer’s disease .
Safety and Toxicology
The safety profile of adenosine derivatives is crucial for their therapeutic application. Preliminary studies indicate that while some derivatives exhibit beneficial effects on cardiovascular function, they must be evaluated for potential side effects such as hypotension or excessive sedation .
Properties
CAS No. |
119631-05-9 |
---|---|
Molecular Formula |
C17H19ClN6O4 |
Molecular Weight |
406.8 g/mol |
IUPAC Name |
(2R,3S,5R)-5-[6-amino-8-[2-chloro-4-(hydroxymethyl)anilino]purin-9-yl]-2-(hydroxymethyl)oxolan-3-ol |
InChI |
InChI=1S/C17H19ClN6O4/c18-9-3-8(5-25)1-2-10(9)22-17-23-14-15(19)20-7-21-16(14)24(17)13-4-11(27)12(6-26)28-13/h1-3,7,11-13,25-27H,4-6H2,(H,22,23)(H2,19,20,21)/t11-,12+,13+/m0/s1 |
InChI Key |
SDLGUEFJMNQSAG-YNEHKIRRSA-N |
Isomeric SMILES |
C1[C@@H]([C@H](O[C@H]1N2C3=NC=NC(=C3N=C2NC4=C(C=C(C=C4)CO)Cl)N)CO)O |
Canonical SMILES |
C1C(C(OC1N2C3=NC=NC(=C3N=C2NC4=C(C=C(C=C4)CO)Cl)N)CO)O |
Origin of Product |
United States |
Disclaimer and Information on In-Vitro Research Products
Please be aware that all articles and product information presented on BenchChem are intended solely for informational purposes. The products available for purchase on BenchChem are specifically designed for in-vitro studies, which are conducted outside of living organisms. In-vitro studies, derived from the Latin term "in glass," involve experiments performed in controlled laboratory settings using cells or tissues. It is important to note that these products are not categorized as medicines or drugs, and they have not received approval from the FDA for the prevention, treatment, or cure of any medical condition, ailment, or disease. We must emphasize that any form of bodily introduction of these products into humans or animals is strictly prohibited by law. It is essential to adhere to these guidelines to ensure compliance with legal and ethical standards in research and experimentation.