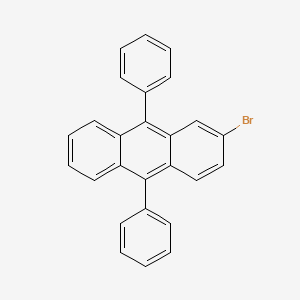
2-Bromo-9,10-diphenylanthracene
Overview
Description
2-Bromo-9,10-diphenylanthracene (CAS: 201731-79-5, molecular formula: C₂₆H₁₇Br) is a brominated derivative of 9,10-diphenylanthracene, featuring a bromine atom at the 2-position of the anthracene core. This compound is characterized by a molecular weight of 409.32 g/mol and a density of 1.344 g/cm³ . It serves as a critical intermediate in synthesizing optoelectronic materials, particularly for organic light-emitting diodes (OLEDs) and phosphorescent complexes. Its synthetic utility stems from the bromine atom’s ability to undergo cross-coupling reactions, enabling the introduction of functional groups such as phosphinoyl or aryl moieties .
Preparation Methods
Bromination of 9,10-Diphenylanthracene
This method involves the direct bromination of 9,10-diphenylanthracene using bromine or N-bromosuccinimide (NBS). The reaction can be catalyzed by iron or copper powder to enhance yield.
- Dissolve 9,10-diphenylanthracene in an organic solvent such as chloroform or dichloromethane.
- Add bromine or NBS gradually while maintaining the reaction temperature.
- Stir the mixture at room temperature or slightly elevated temperatures for several hours.
- After completion, quench the reaction and extract the product using a suitable organic solvent.
Yield and Purity:
This method typically yields high-purity 2-Bromo-9,10-diphenylanthracene with purities exceeding 95% under optimized conditions.
Palladium-Catalyzed Cross-Coupling Reactions
Another effective method for synthesizing this compound involves palladium-catalyzed cross-coupling reactions, particularly using a metal phenyl compound.
- Start with a 9,10-dihalogenoanthracene (e.g., 9,10-dibromoanthracene).
- Combine it with a phenyl compound in the presence of a palladium catalyst (e.g., Pd(PPh₃)₂Cl₂) and a base.
- Conduct the reaction in a solvent such as tetrahydrofuran (THF) under reflux conditions.
- After the reaction period (typically 1 hour), work up the reaction mixture to isolate the product.
Yield and Purity:
This method can achieve yields of around 70-87%, depending on the specific conditions employed.
Alternative Synthesis via Diels-Alder Reaction
A more complex synthesis route involves utilizing a Diels-Alder reaction followed by subsequent transformations.
- React benzoylhydrazide with benzoyl formic acid to form an intermediate.
- Condense this intermediate under dehydration conditions using dicyclohexyl carbodiimide (DCC).
- Perform a Diels-Alder reaction with benzyne to produce the desired compound.
Yield and Purity:
While this method offers an interesting synthetic pathway, it often results in lower yields (around 50%) and requires more steps compared to other methods.
The following table summarizes key aspects of each preparation method for this compound:
Method | Yield (%) | Purity (%) | Key Features |
---|---|---|---|
Bromination | >95 | >95 | Simple and direct; high yield |
Palladium-Catalyzed Coupling | 70-87 | >95 | Efficient for industrial applications |
Diels-Alder Reaction | ~50 | Variable | More complex; lower yield |
Chemical Reactions Analysis
Types of Reactions
2-Bromo-9,10-diphenylanthracene can undergo various chemical reactions, including:
Substitution Reactions: The bromine atom can be substituted with other functional groups using nucleophilic substitution reactions.
Oxidation Reactions: The anthracene core can be oxidized to form anthraquinone derivatives.
Reduction Reactions: The compound can be reduced to form dihydro derivatives.
Common Reagents and Conditions
Substitution Reactions: Common reagents include nucleophiles such as sodium methoxide or potassium tert-butoxide in polar aprotic solvents like dimethyl sulfoxide.
Oxidation Reactions: Oxidizing agents such as potassium permanganate or chromium trioxide in acidic conditions.
Reduction Reactions: Reducing agents like lithium aluminum hydride or sodium borohydride in anhydrous solvents.
Major Products Formed
Substitution Reactions: Products include various substituted anthracenes depending on the nucleophile used.
Oxidation Reactions: Major products are anthraquinone derivatives.
Reduction Reactions: Major products are dihydroanthracene derivatives.
Scientific Research Applications
2-Bromo-9,10-diphenylanthracene has several applications in scientific research:
Chemistry: Used as a precursor in the synthesis of more complex organic molecules and as a reagent in photophysical studies.
Biology: Employed in the study of biological systems due to its fluorescence properties.
Medicine: Investigated for potential use in photodynamic therapy and as a fluorescent probe for imaging.
Mechanism of Action
The mechanism of action of 2-Bromo-9,10-diphenylanthracene is primarily related to its photophysical properties. When exposed to light, the compound can absorb photons and transition to an excited state. This excited state can then transfer energy to other molecules or emit light as fluorescence. The molecular targets and pathways involved include interactions with other chromophores and energy transfer processes in photophysical applications .
Comparison with Similar Compounds
Comparison with Structurally Similar Compounds
Key Observations :
- Substituent Position : Bromination at C2 (vs. C7 or C9) influences reactivity in cross-coupling reactions. For example, 2-bromo derivatives are more reactive in Pd-catalyzed C–P bond formation , while 7-bromo derivatives couple efficiently with δ-carboline .
- Heteroatom Introduction : Replacing C2 with nitrogen (2-aza analogue) simplifies synthesis and induces a 50 nm red shift in OLED emission due to altered HOMO-LUMO levels .
Photophysical and Electrochemical Properties
Table 2: Photophysical and Electronic Properties
Key Findings :
- Red-Shifted Emission: Bromination at C2 induces a 30–50 nm red shift in fluorescence compared to non-brominated 9,10-diphenylanthracene due to enhanced spin-orbit coupling (SOC) .
- Heavy Atom Effect : Bromine promotes intersystem crossing (ISC), enabling room-temperature phosphorescence (RTP) in crystalline and amorphous states .
- Heteroatom Impact : Nitrogen incorporation reduces the HOMO-LUMO gap by 0.6 eV, significantly altering charge transport properties in OLEDs .
Thermal and Stability Profiles
Table 3: Thermal Stability and Purity
Notes:
Biological Activity
2-Bromo-9,10-diphenylanthracene (Br-DPA) is a significant organic compound known for its diverse applications in organic electronics, materials science, and medicinal chemistry. Its biological activity has garnered attention due to its interactions with various biological macromolecules and potential therapeutic applications. This article explores the biochemical properties, mechanisms of action, and research findings related to Br-DPA, supported by data tables and relevant case studies.
Br-DPA has a molecular formula of C26H17Br and a molecular weight of approximately 433.36 g/mol. It is characterized by a large conjugated system that enhances its electronic properties. The compound appears as a white to light yellow crystalline powder with a melting point between 263-265°C. It is insoluble in water but soluble in organic solvents such as chloroform and toluene .
Biochemical Interactions
Br-DPA exhibits significant biochemical activity primarily through its interactions with proteins, enzymes, and nucleic acids:
- Enzyme Interaction: Br-DPA can bind to cytochrome P450 enzymes, influencing their catalytic activity through π-π stacking and hydrophobic interactions. This interaction can modulate the metabolism of various substrates, impacting drug metabolism and toxicity .
- Nucleic Acid Binding: The compound has shown the ability to intercalate with DNA and RNA, potentially affecting gene expression and replication processes. This property makes it a candidate for further investigation in cancer research due to its ability to influence cellular proliferation and apoptosis .
Cellular Effects
Br-DPA's biological activity extends to its effects on cellular processes:
- Oxidative Stress: The compound can generate reactive oxygen species (ROS), leading to oxidative stress within cells. This can result in altered gene expression and metabolic pathways, contributing to both therapeutic effects and toxicity depending on dosage .
- Dose-Dependent Effects: Research indicates that low doses of Br-DPA can modulate cellular functions without significant toxicity, while high doses may induce inflammation and tissue damage due to excessive oxidative stress .
Case Studies
-
Cancer Research:
A study investigated the effects of Br-DPA on cancer cell lines, revealing that it could inhibit cell proliferation at specific concentrations while inducing apoptosis through ROS generation. The results suggest potential applications in targeted cancer therapies. -
Organic Electronics:
Br-DPA is widely utilized in the development of organic light-emitting diodes (OLEDs) due to its excellent electronic properties. Its role as an electron donor enhances the optoelectronic characteristics of devices, making it valuable in material science .
Biological Activity Summary
Activity Type | Description |
---|---|
Enzyme Interaction | Modulates cytochrome P450 activity; affects drug metabolism |
Nucleic Acid Binding | Intercalates with DNA/RNA; influences gene expression |
Oxidative Stress | Generates ROS; leads to oxidative damage at high concentrations |
Cell Proliferation | Inhibits proliferation in cancer cell lines; induces apoptosis |
Dosage Effects on Cellular Function
Dosage Range (µM) | Cellular Response | Observations |
---|---|---|
Low (1-10) | Modulation without toxicity | Enhanced metabolic activity |
Moderate (10-50) | Induction of oxidative stress | Altered gene expression |
High (>50) | Toxicity observed | Inflammation and tissue damage |
Q & A
Basic Research Questions
Q. What are the key synthetic routes for preparing 2-Bromo-9,10-diphenylanthracene, and what factors influence reaction efficiency?
- Methodological Answer : A common synthesis involves reacting 2-bromoanthracene derivatives with phenyl lithium in acetonitrile at −78°C. The reaction requires precise stoichiometry (2.1 equiv. phenyl lithium) and quenching with saturated NH₄Cl to terminate the reaction. Post-synthesis, crude residues are extracted with ethyl acetate, washed with brine, and dried over Na₂SO₄. Purification is typically omitted for immediate use in subsequent steps . Key factors include temperature control (<−70°C), solvent polarity, and exclusion of moisture to prevent side reactions.
Q. How should researchers handle and store this compound to ensure safety and stability?
- Methodological Answer : The compound requires storage in airtight containers under inert gas (e.g., nitrogen) at 0–6°C to prevent degradation. Personal protective equipment (PPE) such as nitrile gloves, N95 respirators, and eye shields is mandatory due to potential skin/eye irritation . Avoid contact with water or heat sources, as decomposition may release hazardous vapors. Spills should be neutralized with non-combustible absorbents (e.g., vermiculite) and disposed of as halogenated waste .
Q. What analytical techniques are recommended for characterizing purity and structural integrity?
- Methodological Answer :
- Nuclear Magnetic Resonance (NMR) : ¹H NMR in CDCl₃ resolves aromatic proton environments (δ 8.60–7.66 ppm for anthracene backbone) .
- High-Performance Liquid Chromatography (HPLC) : Purity assessment (>95% GC) using reverse-phase columns with UV detection at 254 nm .
- X-Ray Crystallography : Single-crystal diffraction confirms molecular geometry (e.g., dihedral angles between phenyl substituents and anthracene core) .
Advanced Research Questions
Q. How does the bromine substituent influence electronic properties compared to non-brominated analogs?
- Methodological Answer : The electron-withdrawing bromine atom stabilizes the LUMO, reducing the reduction potential. Cyclic voltammetry (DMF, Pt electrode) shows a 0.3 V cathodic shift in the first reduction wave compared to 9,10-diphenylanthracene. Computational studies (DFT, B3LYP/6-31G*) correlate this with enhanced electron affinity, critical for charge transport in organic semiconductors .
Q. What contradictions exist in thermodynamic data, and how can they be resolved?
- Methodological Answer : Reported heats of sublimation (ΔHₐ) vary between 120–135 kJ/mol due to differing measurement techniques (e.g., vapor pressure vs. calorimetry). To resolve discrepancies, researchers should standardize conditions (e.g., 25°C, inert atmosphere) and cross-validate using thermogravimetric analysis (TGA) coupled with mass spectrometry .
Q. How can bromination of 9,10-diphenylanthracene be optimized to minimize di-brominated by-products?
- Methodological Answer : Use bromodimethylsulfonium bromide (BDMS) in dichloromethane at room temperature. This reagent avoids toxic Br₂ gas and reduces over-bromination. Reaction monitoring via TLC (hexane:EtOAc 9:1) ensures selective mono-bromination. Yields >85% are achievable with 2.4 equiv. BDMS and NaOH traps for HBr neutralization .
Q. What role does this compound play in OLED material development?
- Methodological Answer : As a blue-emitting dopant, it enhances electroluminescence efficiency. Device performance is assessed via:
- Photoluminescence Quantum Yield (PLQY) : Measured using integrating spheres (e.g., 60–70% in PMMA films).
- Thin-Film Morphology : Atomic force microscopy (AFM) ensures uniform layer deposition (<1 nm roughness) for reduced exciton quenching .
Q. How does electrochemical reduction behavior compare to non-brominated analogs?
- Methodological Answer : In dimethylformamide (DMF), the radical anion (DPA⁻•) of this compound exhibits a 1-electron reversible wave at −2.1 V (vs. Ag/AgCl), whereas non-brominated DPA reduces at −1.8 V. Chronoamperometry reveals slower electron transfer kinetics (k₀ = 0.02 cm/s) due to bromine’s steric and electronic effects, impacting charge transport in optoelectronic devices .
Properties
IUPAC Name |
2-bromo-9,10-diphenylanthracene | |
---|---|---|
Source | PubChem | |
URL | https://pubchem.ncbi.nlm.nih.gov | |
Description | Data deposited in or computed by PubChem | |
InChI |
InChI=1S/C26H17Br/c27-20-15-16-23-24(17-20)26(19-11-5-2-6-12-19)22-14-8-7-13-21(22)25(23)18-9-3-1-4-10-18/h1-17H | |
Source | PubChem | |
URL | https://pubchem.ncbi.nlm.nih.gov | |
Description | Data deposited in or computed by PubChem | |
InChI Key |
OZNXPZBQVNNJCS-UHFFFAOYSA-N | |
Source | PubChem | |
URL | https://pubchem.ncbi.nlm.nih.gov | |
Description | Data deposited in or computed by PubChem | |
Canonical SMILES |
C1=CC=C(C=C1)C2=C3C=CC(=CC3=C(C4=CC=CC=C42)C5=CC=CC=C5)Br | |
Source | PubChem | |
URL | https://pubchem.ncbi.nlm.nih.gov | |
Description | Data deposited in or computed by PubChem | |
Molecular Formula |
C26H17Br | |
Source | PubChem | |
URL | https://pubchem.ncbi.nlm.nih.gov | |
Description | Data deposited in or computed by PubChem | |
DSSTOX Substance ID |
DTXSID30623822 | |
Record name | 2-Bromo-9,10-diphenylanthracene | |
Source | EPA DSSTox | |
URL | https://comptox.epa.gov/dashboard/DTXSID30623822 | |
Description | DSSTox provides a high quality public chemistry resource for supporting improved predictive toxicology. | |
Molecular Weight |
409.3 g/mol | |
Source | PubChem | |
URL | https://pubchem.ncbi.nlm.nih.gov | |
Description | Data deposited in or computed by PubChem | |
CAS No. |
201731-79-5 | |
Record name | 2-Bromo-9,10-diphenylanthracene | |
Source | EPA DSSTox | |
URL | https://comptox.epa.gov/dashboard/DTXSID30623822 | |
Description | DSSTox provides a high quality public chemistry resource for supporting improved predictive toxicology. | |
Synthesis routes and methods I
Procedure details
Synthesis routes and methods II
Procedure details
Retrosynthesis Analysis
AI-Powered Synthesis Planning: Our tool employs the Template_relevance Pistachio, Template_relevance Bkms_metabolic, Template_relevance Pistachio_ringbreaker, Template_relevance Reaxys, Template_relevance Reaxys_biocatalysis model, leveraging a vast database of chemical reactions to predict feasible synthetic routes.
One-Step Synthesis Focus: Specifically designed for one-step synthesis, it provides concise and direct routes for your target compounds, streamlining the synthesis process.
Accurate Predictions: Utilizing the extensive PISTACHIO, BKMS_METABOLIC, PISTACHIO_RINGBREAKER, REAXYS, REAXYS_BIOCATALYSIS database, our tool offers high-accuracy predictions, reflecting the latest in chemical research and data.
Strategy Settings
Precursor scoring | Relevance Heuristic |
---|---|
Min. plausibility | 0.01 |
Model | Template_relevance |
Template Set | Pistachio/Bkms_metabolic/Pistachio_ringbreaker/Reaxys/Reaxys_biocatalysis |
Top-N result to add to graph | 6 |
Feasible Synthetic Routes
Disclaimer and Information on In-Vitro Research Products
Please be aware that all articles and product information presented on BenchChem are intended solely for informational purposes. The products available for purchase on BenchChem are specifically designed for in-vitro studies, which are conducted outside of living organisms. In-vitro studies, derived from the Latin term "in glass," involve experiments performed in controlled laboratory settings using cells or tissues. It is important to note that these products are not categorized as medicines or drugs, and they have not received approval from the FDA for the prevention, treatment, or cure of any medical condition, ailment, or disease. We must emphasize that any form of bodily introduction of these products into humans or animals is strictly prohibited by law. It is essential to adhere to these guidelines to ensure compliance with legal and ethical standards in research and experimentation.