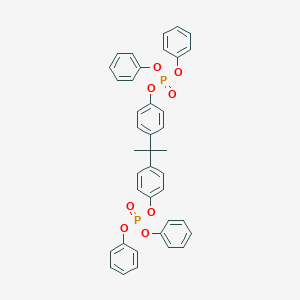
Bisphenol A bis(diphenyl phosphate)
Overview
Description
Bisphenol A bis(diphenyl phosphate) is a well-known flame retardant used in normally flammable resins and is especially useful in flame retarding polycarbonate/acrylonitrile-butadiene-styrene (PC/ABS) compositions . It is also used in electrical wire covering and other flame-resistant materials .
Synthesis Analysis
Bisphenol A bis(diphenyl phosphate) has been synthesized through a simple and green method via melt polycondensation . The process involves the melt polycondensation of phenylphosphonic dichloride and bisphenol A . The synthesized product has been extensively characterized by FTIR, 1H NMR, and 31P NMR .Molecular Structure Analysis
The molecular formula of Bisphenol A bis(diphenyl phosphate) is C39H34O8P2 . It has an average mass of 692.630 Da and a monoisotopic mass of 692.172913 Da .Chemical Reactions Analysis
Bisphenol A bis(diphenyl phosphate) is formed by the transesterification of bisphenol A with triphenyl phosphate . The commercial-grade material can contain oligomers . The reaction and the products provide an effective pathway for bis(diphenyl phosphate) salts to enter the gas phase or act in the condensed phase .Physical And Chemical Properties Analysis
Bisphenol A bis(diphenyl phosphate) is a colorless solid that is soluble in most common organic solvents but has very poor solubility in water .Scientific Research Applications
Flame Retardancy in Polymer Blends
Bisphenol A bis(diphenyl phosphate), commonly known as Fyrolflex BDP, is utilized as a halogen-free flame retardant in various plastics. It is particularly used in polymer blends of engineering plastics like PPO/HIPS and PC/ABS, which are frequently employed to manufacture casings for electrical items such as TVs, computers, and home appliances. The compound is synthesized through the transesterification of bisphenol A with triphenyl phosphate .
Microencapsulation for Compatibility Enhancement
In efforts to improve the compatibility of oil-based flame retardants with waterborne substrates, Fyrolflex BDP is used as the dispersed phase in the preparation of stable oil-in-water (O/W) Pickering emulsions. This process involves using sodium alginate (SA) solution as the continuous phase and hydrophilic silica as the emulsifier .
Lubrication Improvement in Greases
Fyrolflex BDP exhibits tribological characteristics that make it an effective antiwear additive in lubricants such as polyalkylene glycol and polyurea grease. Its inclusion in these substances significantly enhances their lubrication properties .
Biotransformation Studies
The compound has been the subject of biotransformation studies to understand its metabolic pathways. Research involving Wistar-Han rat and herring gull liver-based microsomal assays has been conducted to characterize the in vitro biotransformation of Fyrolflex BDP, with a focus on hypothesized target metabolites like bisphenol-A (BPA) and diphenyl phosphate (DPHP) .
Developmental Toxicity Research
There is growing interest in the developmental toxicity of Fyrolflex BDP, especially concerning the intergenerational effects of parental exposure. Studies using model organisms such as zebrafish have been conducted to explore the potential developmental impacts of exposure to various concentrations of this compound .
Safety And Hazards
properties
IUPAC Name |
[4-[2-(4-diphenoxyphosphoryloxyphenyl)propan-2-yl]phenyl] diphenyl phosphate | |
---|---|---|
Source | PubChem | |
URL | https://pubchem.ncbi.nlm.nih.gov | |
Description | Data deposited in or computed by PubChem | |
InChI |
InChI=1S/C39H34O8P2/c1-39(2,31-23-27-37(28-24-31)46-48(40,42-33-15-7-3-8-16-33)43-34-17-9-4-10-18-34)32-25-29-38(30-26-32)47-49(41,44-35-19-11-5-12-20-35)45-36-21-13-6-14-22-36/h3-30H,1-2H3 | |
Source | PubChem | |
URL | https://pubchem.ncbi.nlm.nih.gov | |
Description | Data deposited in or computed by PubChem | |
InChI Key |
BQPNUOYXSVUVMY-UHFFFAOYSA-N | |
Source | PubChem | |
URL | https://pubchem.ncbi.nlm.nih.gov | |
Description | Data deposited in or computed by PubChem | |
Canonical SMILES |
CC(C)(C1=CC=C(C=C1)OP(=O)(OC2=CC=CC=C2)OC3=CC=CC=C3)C4=CC=C(C=C4)OP(=O)(OC5=CC=CC=C5)OC6=CC=CC=C6 | |
Source | PubChem | |
URL | https://pubchem.ncbi.nlm.nih.gov | |
Description | Data deposited in or computed by PubChem | |
Molecular Formula |
C39H34O8P2 | |
Source | PubChem | |
URL | https://pubchem.ncbi.nlm.nih.gov | |
Description | Data deposited in or computed by PubChem | |
DSSTOX Substance ID |
DTXSID8052720 | |
Record name | 2,2-Bis[4-[bis(phenoxy)phosphoryloxy]phenyl]propane | |
Source | EPA DSSTox | |
URL | https://comptox.epa.gov/dashboard/DTXSID8052720 | |
Description | DSSTox provides a high quality public chemistry resource for supporting improved predictive toxicology. | |
Molecular Weight |
692.6 g/mol | |
Source | PubChem | |
URL | https://pubchem.ncbi.nlm.nih.gov | |
Description | Data deposited in or computed by PubChem | |
Physical Description |
Liquid; Other Solid; Pellets or Large Crystals | |
Record name | Phosphoric acid, P,P'-[(1-methylethylidene)di-4,1-phenylene] P,P,P',P'-tetraphenyl ester | |
Source | EPA Chemicals under the TSCA | |
URL | https://www.epa.gov/chemicals-under-tsca | |
Description | EPA Chemicals under the Toxic Substances Control Act (TSCA) collection contains information on chemicals and their regulations under TSCA, including non-confidential content from the TSCA Chemical Substance Inventory and Chemical Data Reporting. | |
Product Name |
Bisphenol A bis(diphenyl phosphate) | |
CAS RN |
5945-33-5 | |
Record name | Bisphenol A bis(diphenyl phosphate) | |
Source | CAS Common Chemistry | |
URL | https://commonchemistry.cas.org/detail?cas_rn=5945-33-5 | |
Description | CAS Common Chemistry is an open community resource for accessing chemical information. Nearly 500,000 chemical substances from CAS REGISTRY cover areas of community interest, including common and frequently regulated chemicals, and those relevant to high school and undergraduate chemistry classes. This chemical information, curated by our expert scientists, is provided in alignment with our mission as a division of the American Chemical Society. | |
Explanation | The data from CAS Common Chemistry is provided under a CC-BY-NC 4.0 license, unless otherwise stated. | |
Record name | Bisphenol A tetraphenyl diphosphate | |
Source | ChemIDplus | |
URL | https://pubchem.ncbi.nlm.nih.gov/substance/?source=chemidplus&sourceid=0005945335 | |
Description | ChemIDplus is a free, web search system that provides access to the structure and nomenclature authority files used for the identification of chemical substances cited in National Library of Medicine (NLM) databases, including the TOXNET system. | |
Record name | Phosphoric acid, P,P'-[(1-methylethylidene)di-4,1-phenylene] P,P,P',P'-tetraphenyl ester | |
Source | EPA Chemicals under the TSCA | |
URL | https://www.epa.gov/chemicals-under-tsca | |
Description | EPA Chemicals under the Toxic Substances Control Act (TSCA) collection contains information on chemicals and their regulations under TSCA, including non-confidential content from the TSCA Chemical Substance Inventory and Chemical Data Reporting. | |
Record name | 2,2-Bis[4-[bis(phenoxy)phosphoryloxy]phenyl]propane | |
Source | EPA DSSTox | |
URL | https://comptox.epa.gov/dashboard/DTXSID8052720 | |
Description | DSSTox provides a high quality public chemistry resource for supporting improved predictive toxicology. | |
Record name | (1-methylethylidene)di-4,1-phenylene tetraphenyl diphosphate | |
Source | European Chemicals Agency (ECHA) | |
URL | https://echa.europa.eu/substance-information/-/substanceinfo/100.102.368 | |
Description | The European Chemicals Agency (ECHA) is an agency of the European Union which is the driving force among regulatory authorities in implementing the EU's groundbreaking chemicals legislation for the benefit of human health and the environment as well as for innovation and competitiveness. | |
Explanation | Use of the information, documents and data from the ECHA website is subject to the terms and conditions of this Legal Notice, and subject to other binding limitations provided for under applicable law, the information, documents and data made available on the ECHA website may be reproduced, distributed and/or used, totally or in part, for non-commercial purposes provided that ECHA is acknowledged as the source: "Source: European Chemicals Agency, http://echa.europa.eu/". Such acknowledgement must be included in each copy of the material. ECHA permits and encourages organisations and individuals to create links to the ECHA website under the following cumulative conditions: Links can only be made to webpages that provide a link to the Legal Notice page. | |
Record name | Phosphoric acid, P,P'-[(1-methylethylidene)di-4,1-phenylene] P,P,P',P'-tetraphenyl ester | |
Source | European Chemicals Agency (ECHA) | |
URL | https://echa.europa.eu/substance-information/-/substanceinfo/100.123.794 | |
Description | The European Chemicals Agency (ECHA) is an agency of the European Union which is the driving force among regulatory authorities in implementing the EU's groundbreaking chemicals legislation for the benefit of human health and the environment as well as for innovation and competitiveness. | |
Explanation | Use of the information, documents and data from the ECHA website is subject to the terms and conditions of this Legal Notice, and subject to other binding limitations provided for under applicable law, the information, documents and data made available on the ECHA website may be reproduced, distributed and/or used, totally or in part, for non-commercial purposes provided that ECHA is acknowledged as the source: "Source: European Chemicals Agency, http://echa.europa.eu/". Such acknowledgement must be included in each copy of the material. ECHA permits and encourages organisations and individuals to create links to the ECHA website under the following cumulative conditions: Links can only be made to webpages that provide a link to the Legal Notice page. | |
Synthesis routes and methods
Procedure details
Retrosynthesis Analysis
AI-Powered Synthesis Planning: Our tool employs the Template_relevance Pistachio, Template_relevance Bkms_metabolic, Template_relevance Pistachio_ringbreaker, Template_relevance Reaxys, Template_relevance Reaxys_biocatalysis model, leveraging a vast database of chemical reactions to predict feasible synthetic routes.
One-Step Synthesis Focus: Specifically designed for one-step synthesis, it provides concise and direct routes for your target compounds, streamlining the synthesis process.
Accurate Predictions: Utilizing the extensive PISTACHIO, BKMS_METABOLIC, PISTACHIO_RINGBREAKER, REAXYS, REAXYS_BIOCATALYSIS database, our tool offers high-accuracy predictions, reflecting the latest in chemical research and data.
Strategy Settings
Precursor scoring | Relevance Heuristic |
---|---|
Min. plausibility | 0.01 |
Model | Template_relevance |
Template Set | Pistachio/Bkms_metabolic/Pistachio_ringbreaker/Reaxys/Reaxys_biocatalysis |
Top-N result to add to graph | 6 |
Feasible Synthetic Routes
Disclaimer and Information on In-Vitro Research Products
Please be aware that all articles and product information presented on BenchChem are intended solely for informational purposes. The products available for purchase on BenchChem are specifically designed for in-vitro studies, which are conducted outside of living organisms. In-vitro studies, derived from the Latin term "in glass," involve experiments performed in controlled laboratory settings using cells or tissues. It is important to note that these products are not categorized as medicines or drugs, and they have not received approval from the FDA for the prevention, treatment, or cure of any medical condition, ailment, or disease. We must emphasize that any form of bodily introduction of these products into humans or animals is strictly prohibited by law. It is essential to adhere to these guidelines to ensure compliance with legal and ethical standards in research and experimentation.