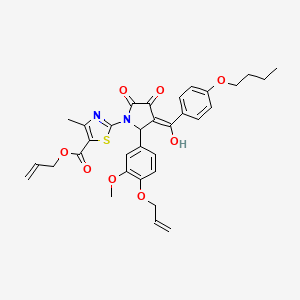
Allyl 2-(2-(4-(allyloxy)-3-methoxyphenyl)-3-(4-butoxybenzoyl)-4-hydroxy-5-oxo-2,5-dihydro-1H-pyrrol-1-yl)-4-methylthiazole-5-carboxylate
- Click on QUICK INQUIRY to receive a quote from our team of experts.
- With the quality product at a COMPETITIVE price, you can focus more on your research.
Overview
Description
The compound, with the molecular formula C₃₃H₃₆N₂O₇S (monoisotopic mass: 604.224322), features a complex heterocyclic framework combining a pyrrolidine-dione core fused with a thiazole ring and multiple aromatic substituents . Key structural elements include:
- Pyrrol-dihydrothiazole scaffold: A 2,5-dihydro-1H-pyrrol-1-yl moiety linked to a 4-methylthiazole-5-carboxylate group.
- Functional groups: Allyl ester, hydroxyl, and ketone groups, which may influence solubility, reactivity, and intermolecular hydrogen bonding.
Preparation Methods
Synthetic Routes and Reaction Conditions
The synthesis of Allyl 2-(2-(4-(allyloxy)-3-methoxyphenyl)-3-(4-butoxybenzoyl)-4-hydroxy-5-oxo-2,5-dihydro-1H-pyrrol-1-yl)-4-methylthiazole-5-carboxylate involves multiple steps, each requiring specific reagents and conditions. The process typically begins with the preparation of intermediate compounds, which are then combined through a series of reactions to form the final product. Common reagents used in these reactions include allyl bromide, methoxyphenyl derivatives, butoxybenzoyl chloride, and various catalysts to facilitate the reactions.
Industrial Production Methods
Industrial production of this compound would likely involve scaling up the laboratory synthesis methods to accommodate larger quantities. This would require optimization of reaction conditions, such as temperature, pressure, and solvent choice, to ensure high yield and purity. Additionally, industrial processes would need to consider factors such as cost, safety, and environmental impact.
Chemical Reactions Analysis
Types of Reactions
Allyl 2-(2-(4-(allyloxy)-3-methoxyphenyl)-3-(4-butoxybenzoyl)-4-hydroxy-5-oxo-2,5-dihydro-1H-pyrrol-1-yl)-4-methylthiazole-5-carboxylate can undergo various chemical reactions, including:
Oxidation: This reaction involves the addition of oxygen or the removal of hydrogen, often using oxidizing agents such as potassium permanganate or hydrogen peroxide.
Reduction: This reaction involves the addition of hydrogen or the removal of oxygen, typically using reducing agents like sodium borohydride or lithium aluminum hydride.
Substitution: This reaction involves the replacement of one functional group with another, often using reagents such as halogens or nucleophiles.
Common Reagents and Conditions
Common reagents used in the reactions of this compound include oxidizing agents (e.g., potassium permanganate), reducing agents (e.g., sodium borohydride), and various nucleophiles and electrophiles. Reaction conditions such as temperature, solvent, and pH must be carefully controlled to achieve the desired outcome.
Major Products Formed
The major products formed from these reactions depend on the specific conditions and reagents used. For example, oxidation may yield carboxylic acids or ketones, while reduction may produce alcohols or amines. Substitution reactions can result in a wide range of products, depending on the nature of the substituent.
Scientific Research Applications
Chemistry: This compound can be used as a building block for the synthesis of more complex molecules, as well as a reagent in various organic reactions.
Biology: Its unique structure may allow it to interact with biological molecules, making it a candidate for studies on enzyme inhibition or receptor binding.
Medicine: The compound’s potential biological activity could lead to its use in drug development, particularly for targeting specific pathways or diseases.
Industry: Its chemical properties may make it useful in the development of new materials or as a catalyst in industrial processes.
Mechanism of Action
The mechanism of action of Allyl 2-(2-(4-(allyloxy)-3-methoxyphenyl)-3-(4-butoxybenzoyl)-4-hydroxy-5-oxo-2,5-dihydro-1H-pyrrol-1-yl)-4-methylthiazole-5-carboxylate involves its interaction with specific molecular targets. These targets may include enzymes, receptors, or other proteins, leading to changes in cellular processes. The compound’s structure allows it to bind to these targets, potentially inhibiting or activating their function. The exact pathways involved would depend on the specific biological context and the nature of the target molecules.
Comparison with Similar Compounds
Structural and Electronic Comparisons
The compound’s structural analogs can be categorized based on core scaffolds and substituents:
Key Observations :
Core Scaffold Diversity: The target compound’s pyrrol-dihydrothiazole core differs from thiazole-triazole hybrids () and pyrrol-2-one derivatives (), impacting rigidity and electronic delocalization.
Substituent Effects :
- Electron-Donating Groups : The 4-butoxybenzoyl (target) and 4-isopropylphenyl () groups enhance lipophilicity, whereas 4-fluorophenyl () introduces electron-withdrawing effects.
- Steric Hindrance : The allyloxy and butoxy groups in the target compound may reduce reactivity compared to smaller substituents like chloro or fluoro .
Synthetic Efficiency :
- Thiazole derivatives () were synthesized in high yields using DMF crystallization, whereas pyrrol-2-one derivatives () achieved only 52% yield, highlighting the challenge of introducing bulky substituents .
Electronic and Reactivity Profiles
- Isoelectronicity vs. Isovalency: While the target compound shares electronic similarities with brominated isoxazolidinones (), structural disparities (e.g., lack of a trifluoromethyl group) limit direct comparisons. emphasizes that "isovalency" (similar valency but divergent geometry) better explains differences in properties like catalytic activity or solubility .
Analytical Tools
- Crystallography : SHELXL () and SHELXTL are widely used for refining structures of similar complexity, though the target compound’s crystallographic data remain uncharacterized .
Biological Activity
Allyl 2-(2-(4-(allyloxy)-3-methoxyphenyl)-3-(4-butoxybenzoyl)-4-hydroxy-5-oxo-2,5-dihydro-1H-pyrrol-1-yl)-4-methylthiazole-5-carboxylate is a complex organic compound that combines various functional groups known for their biological activities. This article explores its synthesis, structural characteristics, and potential biological effects, drawing from diverse sources.
Chemical Structure and Synthesis
The compound features a thiazole ring, pyrrole moiety, and multiple allyl and phenolic substituents. These structural elements contribute to its chemical reactivity and potential biological activities. The synthesis typically involves several steps, including the formation of the thiazole and pyrrole rings, followed by functionalization with allyl and butoxy groups.
Synthesis Overview
- Formation of Thiazole Ring : The thiazole component is synthesized via cyclization reactions involving appropriate precursors.
- Pyrrole Formation : The pyrrole ring can be constructed through condensation reactions of suitable amines with carbonyl compounds.
- Allylation and Functionalization : The introduction of allyl groups is achieved through nucleophilic substitutions or allylation reactions using reagents like allyl bromide.
Antimicrobial Properties
Compounds containing thiazole and pyrrole rings are often noted for their antimicrobial activities. For instance, thiazole derivatives have been reported to exhibit significant antibacterial and antifungal properties due to their ability to disrupt microbial cell functions.
Compound Type | Structural Features | Reported Biological Activity |
---|---|---|
Thiazole Derivatives | Thiazole ring | Antimicrobial, anticancer |
Pyrrole Derivatives | Pyrrole ring | Antioxidant, anti-inflammatory |
Allyl Compounds | Allyl group | Precursor in synthesis with potential biological effects |
The specific biological effects of this compound are not extensively documented; however, its structural similarities to known bioactive compounds suggest potential applications in medicinal chemistry.
Case Studies
Recent studies have highlighted the biological activity of structurally similar compounds:
- Eugenol (4-Allyl-2-methoxyphenol) : This compound has shown significant antibiofilm activity against azole-resistant Aspergillus fumigatus. It modulates gene expression related to efflux pumps and biofilm formation, indicating a mechanism that could be relevant for the allyl compound .
- Thiazole Derivatives : Research indicates that thiazole-containing compounds exhibit promising antimicrobial activities against various bacterial strains. Their mechanisms often involve interference with bacterial cell wall synthesis or function.
- Antioxidant Activity : Pyrrole derivatives have been documented for their antioxidant properties, which may contribute to cellular protection against oxidative stress .
Future Directions
Given the complex nature of this compound, further research is warranted to elucidate its full biological profile. Suggested areas for future investigation include:
- In vitro and in vivo studies to assess antimicrobial efficacy.
- Mechanistic studies to understand how the compound interacts with biological targets.
- Pharmacokinetic evaluations to determine absorption, distribution, metabolism, and excretion (ADME) properties.
Q & A
Basic Research Questions
Q. What are the recommended synthetic pathways for this compound, and how do reaction conditions influence yield?
Methodological Answer: Synthesis typically involves multi-step heterocyclization. A general approach includes:
Pyrrole Core Formation : Adapt the Paal-Knorr reaction (condensation of γ-diketones with amines) to construct the dihydro-1H-pyrrol-1-yl moiety, as demonstrated in trifluoromethoxyphenyl-pyrrole synthesis .
Thiazole Ring Assembly : Use chloroacylation followed by cyclization with thioureas (e.g., as in chain heterocyclization for thiazole derivatives) .
Esterification : Introduce the allyl and butoxy groups via nucleophilic substitution or Mitsunobu reactions.
Critical Parameters :
- Reflux Time : Extended reflux (≥4 hours) improves cyclization efficiency but risks thermal degradation .
- Catalyst : Glacial acetic acid enhances imine formation in triazole derivatives, a strategy applicable here .
Q. How should researchers characterize this compound’s purity and structural integrity?
Methodological Answer:
- Spectroscopic Analysis :
- NMR : Use 1H- and 13C-NMR to confirm substitution patterns (e.g., allyloxy, methoxy groups). 19F-NMR is irrelevant here but ^{1H-1H COSY resolves overlapping proton signals in the pyrrole-thiazole core .
- HRMS : Validate molecular weight (expected error <2 ppm).
- Chromatography :
Data Contradictions : Discrepancies in NMR shifts may arise from tautomerism in the dihydro-pyrrole ring. Compare experimental data with DFT-calculated chemical shifts .
Q. What biological activity assays are suitable for this compound?
Methodological Answer:
- In Vitro Screening :
- Enzyme Inhibition : Target kinases or cyclooxygenases (COX-2) due to the thiazole-pyrrole scaffold’s affinity for ATP-binding pockets. Use fluorescence polarization assays .
- Antimicrobial Activity : Follow CLSI guidelines for MIC determination against Gram-positive bacteria (e.g., S. aureus), leveraging the methoxy/butoxy groups’ membrane-disruptive potential .
- Control Experiments : Include reference inhibitors (e.g., staurosporine for kinases) to validate assay conditions .
Advanced Research Questions
Q. How can computational methods predict this compound’s reactivity and stability?
Methodological Answer:
- DFT Calculations :
- Degradation Pathways : Simulate hydrolytic stability (pH 7.4 buffer, 37°C). The ester linkages (allyl carboxylate, butoxybenzoyl) are likely hydrolysis-prone. Use LC-MS to detect degradation products .
Stability Condition | Primary Degradation Product | Half-Life (Predicted) |
---|---|---|
Acidic (pH 2.0) | Free carboxylic acid | 12–24 hours |
Alkaline (pH 9.0) | Demethylated pyrrole | 4–8 hours |
Q. What strategies resolve contradictions in spectroscopic data versus computational predictions?
Methodological Answer:
- Tautomerism Analysis : The 4-hydroxy-5-oxo group may tautomerize, altering NMR shifts. Perform variable-temperature NMR (VT-NMR) to observe equilibrium shifts .
- X-ray Crystallography : Resolve ambiguous NOEs in the thiazole-pyrrole junction. Compare with Cambridge Structural Database entries for similar scaffolds .
- Dynamic NMR Simulations : Use software like MestReNova to model exchange broadening effects .
Q. How can enantioselective synthesis be achieved for stereochemical variants?
Methodological Answer:
- Chiral Auxiliaries : Introduce a menthol-based ester during thiazole formation, later removed via hydrolysis .
- Asymmetric Catalysis : Use Pd-catalyzed allylic alkylation with (R)-BINAP ligands to control stereochemistry at the pyrrole’s 3-position .
- Analytical Validation : Employ chiral HPLC (Chiralpak IA column) with heptane:IPA (90:10) to confirm enantiomeric excess (>98%) .
Q. What process engineering challenges arise in scaling up synthesis?
Methodological Answer:
- Separation Technology : Membrane filtration (e.g., nanofiltration) isolates intermediates without thermal degradation, critical for the acid-labile butoxybenzoyl group .
- Reactor Design : Use microreactors for exothermic steps (e.g., Paal-Knorr reaction) to maintain temperature control and improve yield .
Scale-Up Factor | Challenge | Mitigation Strategy |
---|---|---|
Mixing Efficiency | Heterogeneous reaction during cyclization | Ultrasonic agitation |
Solvent Recovery | Ethanol reflux volume reduction | Thin-film evaporation |
Properties
CAS No. |
609796-66-9 |
---|---|
Molecular Formula |
C33H34N2O8S |
Molecular Weight |
618.7 g/mol |
IUPAC Name |
prop-2-enyl 2-[(3E)-3-[(4-butoxyphenyl)-hydroxymethylidene]-2-(3-methoxy-4-prop-2-enoxyphenyl)-4,5-dioxopyrrolidin-1-yl]-4-methyl-1,3-thiazole-5-carboxylate |
InChI |
InChI=1S/C33H34N2O8S/c1-6-9-18-41-23-13-10-21(11-14-23)28(36)26-27(22-12-15-24(42-16-7-2)25(19-22)40-5)35(31(38)29(26)37)33-34-20(4)30(44-33)32(39)43-17-8-3/h7-8,10-15,19,27,36H,2-3,6,9,16-18H2,1,4-5H3/b28-26+ |
InChI Key |
TXBPFOBFPCPFCW-BYCLXTJYSA-N |
Isomeric SMILES |
CCCCOC1=CC=C(C=C1)/C(=C\2/C(N(C(=O)C2=O)C3=NC(=C(S3)C(=O)OCC=C)C)C4=CC(=C(C=C4)OCC=C)OC)/O |
Canonical SMILES |
CCCCOC1=CC=C(C=C1)C(=C2C(N(C(=O)C2=O)C3=NC(=C(S3)C(=O)OCC=C)C)C4=CC(=C(C=C4)OCC=C)OC)O |
Origin of Product |
United States |
Disclaimer and Information on In-Vitro Research Products
Please be aware that all articles and product information presented on BenchChem are intended solely for informational purposes. The products available for purchase on BenchChem are specifically designed for in-vitro studies, which are conducted outside of living organisms. In-vitro studies, derived from the Latin term "in glass," involve experiments performed in controlled laboratory settings using cells or tissues. It is important to note that these products are not categorized as medicines or drugs, and they have not received approval from the FDA for the prevention, treatment, or cure of any medical condition, ailment, or disease. We must emphasize that any form of bodily introduction of these products into humans or animals is strictly prohibited by law. It is essential to adhere to these guidelines to ensure compliance with legal and ethical standards in research and experimentation.